The first-time detection of Gravitational Waves (GW) by researchers at the Laser Interferometer Gravitational-wave Observatory (LIGO) in 2015 triggered a revolution in astronomy. This phenomenon consists of ripples in spacetime caused by the merger of massive objects and was predicted a century prior by Einstein’s Theory of General Relativity. In the coming years, this burgeoning field will advance considerably thanks to the introduction of next-generation observatories, like the Laser Interferometer Space Antenna (LISA).
With greater sensitivity, astronomers will be able to trace GW events back to their source and use them to probe the interiors of exotic objects and the laws of physics. As part of their Voyage 2050 planning cycle, the European Space Agency (ESA) is considering mission themes that could be ready by 2050 – including GW astronomy. In a recent paper, researchers from the ESA’s Mission Analysis Section and the University of Glasgow presented a new concept that would build on LISA – known as LISAmax. As they report, this observatory could potentially improve GW sensitivity by two orders of magnitude.
The research was led by theoretical physics Dr. Waldemar Martens, a Mission Analyst at the ESA’s European Space Operations Center (ESOC) in Darmstadt, Germany. He was joined by aerospace engineer and astrophysicist Michael Khan, also a Missions Analyst at the ESOC, and astrophysicist Dr. Jean-Baptiste Bayle, an astronomy and astrophysics Research Fellow with the University of Glasgow. The paper that describes their findings appeared online recently and is currently being reviewed for publication by the journal Classical and Quantum Gravity.
Since they were first detected by LIGO scientists in 2015, researchers with LIGO and other observatories worldwide have refined the types of GW events they can detect. This includes the Virgo Observatory in Italy (near Pisa) and the Kamioka Gravitational Wave Detector (KAGRA) in Hida, Japan. These observatories have since partnered with LIGO, forming the Ligo-Virgo-KAGRA (LVK) Collaboration. The efforts of these and other observatories, plus upgrades that have provided increased sensitivity, have multiplied the number of events detected and even traced some back to their sources.
As Dr. Martens told Universe Today via email, this pioneering work has been invaluable. But like all forms of astronomy, future progress is dependent in part on having observatories in space:
“Now that there is no doubt that gravitational waves can be measured, astronomers want to use them as an additional source of information where previously only electromagnetic waves were available. Earth-based detectors, like LIGO/Virgo/Kagra are sensitive in the frequency range of tens of Hertz to several kilo-Hertz. This makes them sensitive to sources like black hole mergers of a few tens of solar masses.
“However, it is known that much larger objects, like super-massive black holes (>10^6 solar masses), exist in the center of galaxies. Mergers of these objects produce gravitational waves far below the sensitive band of Earth-based detectors. To see them, we have to go to space and construct an observatory, like LISA, that has an arm length of 2.5 million km.”
So far, astronomers have detected GW events caused by binary black holes (BBHs) or binary neutron stars (kilonova events), where the co-orbiting bodies eventually merged. It is also theorized that there are many other potential sources, and studying these events could advance our understanding of the Universe. “Among those are primordial gravitational waves that were produced during processes a fraction of a second after the Big Bang,” said Dr. Martens. “We hope that LISA can detect those, but it is not clear yet. That’s one of the reasons why detectors with higher sensitivity and/or different frequency bands are considered for Voyage 2050.”
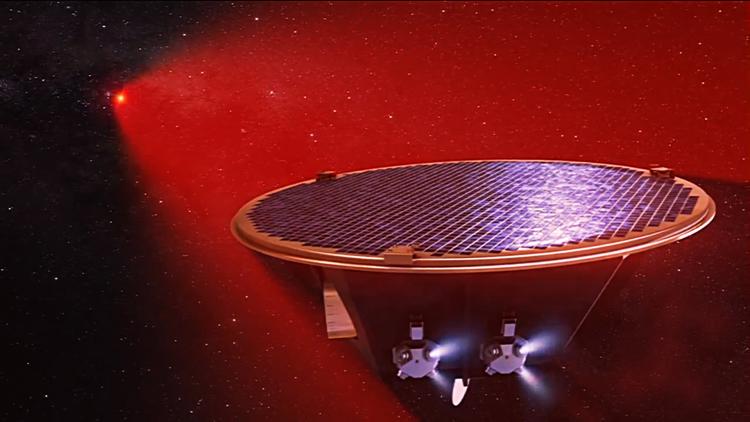
The Voyager 2050 is the latest planning cycle to become part of the agency’s Scientific Program, the foundation and the main “mandatory program” of the European Space Agency. All member states must contribute, and science goals, proposals, and funding are selected by unanimous decision. These cycles aim to set a long-term funding horizon that allows member states to plan their priorities well in advance and provide the European scientific community with a clear vision of what research areas deserve investment and development.
Since the 1980s, the Program has been planned with cycles of approximately 20 years, consistent with the amount of time necessary to prepare ambitious space missions. The first planning cycle (Horizon 2000) was established in 1984 and consisted of decisions that led to the Solar and Heliospheric Observatory (SOHO), Cluster, Rosetta, XMM-Newton, and Herschel missions from the mid-1990 to the early 21st century. In 2005, a further planning cycle (Cosmic Vision) was launched, including mission proposals that would be realized between 2015 and 2025.
This prepared the way for missions like the recently launched JUpiter ICy moons Explorer (JUICE) and the Advanced Telescope for High Energy Astrophysics (ATHENA) X-ray observatory and LISA missions scheduled to launch by the 2030s. The most recent cycle, Voyage 2050, was initiated by the ESA Director of Science Carole Mundell to select scientific properties to follow up on the ATHENA and LISA missions. While these missions will be game-changing, especially in collaboration, Dr. Martens and his colleagues propose ways in which the LISA mission could be enhanced further. As he explained:
“The basic idea of LISAmax is to detect GWs at even lower frequencies than what LISA can do. To be sensitive to these frequencies, one must increase the laser arms of the detector. Larger arms mean larger wavelengths and, thus, lower frequencies. The three LISAmax spacecraft are placed close to the triangular Lagrange points in the Sun-Earth system, which gives the detector an arm length of 259 million km. For comparison, LISA’s arms have a length of 2.5 million km. This makes LISAmax sensitive to GWs in the micro-Hertz band and opens a new window for GW astronomy.
“Generally speaking, whatever source can be measured by LISA below 1 mHz, can be measured with LISAmax at a signal-to-noise ratio that is about two orders of magnitude better. An example that is discussed in the paper is the inspiral phase of supermassive black hole binaries. While LISA will only be able to see such sources shortly before the final merger event, LISAmax can observe these objects thousands of years before, thus allowing for a much better measurement of certain parameters.”

The scientific community is investigating this concept, which could have drastic implications for the future of GW astronomy. In addition to expanding the range of GW events that could be detected, next-generation GW observatories can trace more events back to their sources. On top of that, astronomers anticipate that GWs will allow them to explore the laws of physics, probe the interiors of extreme objects, and even aid in studying planets and satellites.
The proposal put forth by Dr. Martens and his colleagues is one of several GW concepts submitted to the ESA for the Voyage 2050 program. These concepts include a space-based interferometer that would survey the sky for GWs in the millihertz to microhertz (mHz to ?-Hz) frequency range. Another proposes how interferometers sensitive to GWs in the mHz range could be used to learn more about the nature of black holes. Others show how observations in the decihertz (dHz) range could provide the “missing link” for GW astronomy, while high-angular astronomy could help trace GWs back to their source.
Research into the physics of the early Universe, which includes the study of primordial gravitational waves, is also a major theme of the ESA’s Voyage 2050 program. By examining the GWs created during the inflationary epoch, scientists would finally be able to probe the physics and microphysics of this early cosmic period.
Further Reading: arXiv, Voyage 2050
Good news!
“By examining the GWs created during the inflationary epoch, scientists would finally be able to probe the physics and microphysics of this early cosmic period.”
Besides B mode observations, which can be made by other observatories but maybe not these, there are the infaltion decay period. If the inflation field is just right, there can be long-lived decay modes. See e.g. this new result in that direction: “Enhanced Gravitational Waves from Inflaton Oscillons Kaloian D. Lozanov and Volodymyr Takhistov
Phys. Rev. Lett. 130, 181002 – Published 2 May 2023”. “We demonstrate that matter dominance of oscillons, followed by their rapid decay, significantly enhances the primordial gravitational wave (GW) spectrum.”
However, while it is hard to say something on the inflation physics models I don’t think the studied models that approximate power law models bode well for the existence of such decay modes. See e.g. “Squeezing down the Theory Space for Cosmic Inflation” Daniel Meerburg Faculty of Science and Engineering, University of Groningen, Groningen, Netherlands October 4, 2021• Physics 14, 135 on the latest BICEP/Keck data on the cosmic background radiation:
“The value that r takes within a particular inflation model is partly dependent on the amount of expansion before the end of inflation, characterized by the number N of e-folds (one e -fold corresponds to a stretching of space by a factor of e?2.71). To solve the cosmological conundrums that inflation was designed to solve, N has to be at least 40, while values larger than 60 are inconsistent with measurements of n_s . For a large class of popular models broadly referred to as monomial or power-law models, the tensor-to-scalar ratio is relatively large and scales as 1?N [7]. We can now comfortably start to rule out this class of models.” [See the figure in that article, the exclusion is definitive.]