When human beings start living in space for extended periods of time they will need to be as self-sufficient as possible. The same holds true for settlements built on the Moon, on Mars, and other bodies in the Solar System. To avoid being entirely dependent on resupply missions from Earth (which is costly and time-consuming) the inhabitants will need to harvest resources locally – aka. In-Situ Resource Utilization (ISRU).
This means they’ll have to procure their own sources of water, building materials, and grow their own food. While the ISS has allowed for all kinds of experiments involving hydroponics in space, little has been done to see how soil fares in microgravity (or lower gravity). To address this, Morgan Irons – Chief Science Officer of the Virginia-based startup Deep Space Ecology (DSE) – recently sent her Soil Health in Space experiment to the ISS.
The experiment, Determination of Gravitational Effects on Soil Stability for Controlled Environment Agriculture, was sponsored through Morgan’s fellowship with the Norfolk Institute – which provided grant funding from numerous companies (including DSE). An agreement between Norfolk Institute and Rhodium Scientific, LLC (an official space-flight implementation partner of the ISS U.S. National Laboratory) provided access to the NASA launch opportunities and the ISS.
The experiment launched for the ISS on October 2nd, 2020, from NASA’s Wallops Flight Facility near Wattsville, Virginia. As one of several scientific payloads and technology demonstrators, this one has the honor of being the first of its kind to be sent to the ISS. While seeds and plants have been sent to space since the beginning of the Space Age, no Earth soil has ever been launched.
In fact, all previous attempts to study if Earth plants can grow in non-Earth environments have focused on procuring samples of regolith like the Moon, Mars, asteroids, and elsewhere in the Solar System to see if they can be used as soil. And for the most part, experiments have involved simulants rather than the real thing (given the cost of procuring samples of regolith).
This experiment, which examines the effects spaceflight and microgravity have on soil aggregates formed by fungi and bacteria, represents the culmination of years’ worth of work and study by father-daughter duo Lee and Morgan Irons, who co-founded Deep Space Ecology together. As Lee told Universe Today via email:
“Deep Space Ecology is all about food security. Because Mars is the most challenging food security problem, if we can solve the problem for Mars, then we can solve food security problems anywhere on Earth, from below-sea-level salt flats to the high Himalayas, from the Sahara to the Arctic, from the most remote places on Earth to the most dense urban centers.”
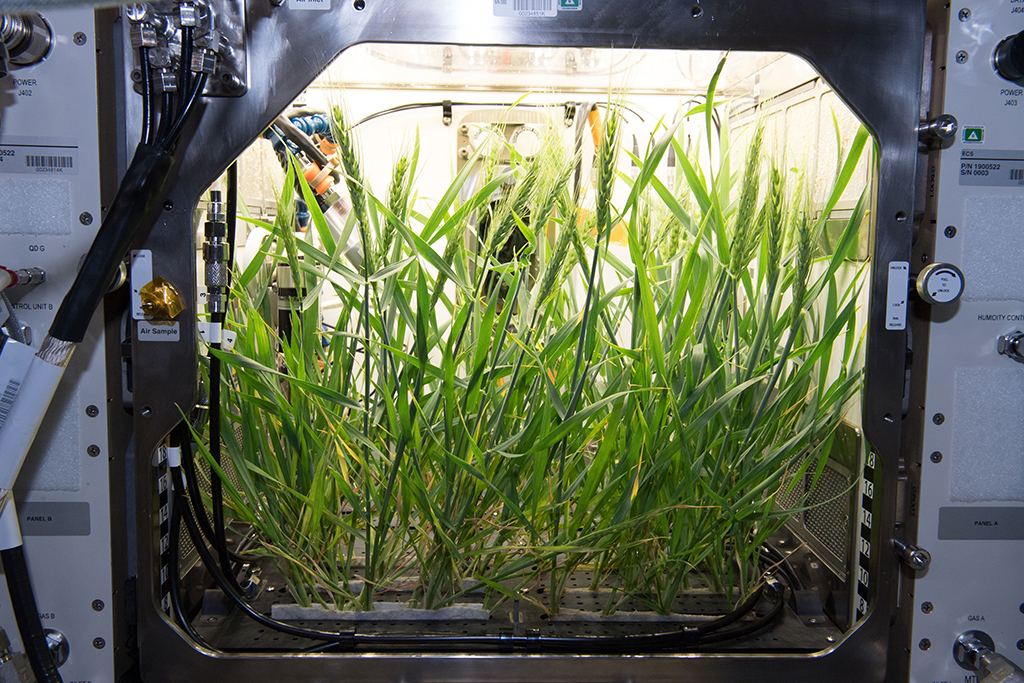
The Right Stuff
The story of DSE began in 2016, shortly after Morgan and Lee attended the Humans to Mars Summit (H2M) in Washington DC. This annual event, hosted by Explore Mars, brings researchers from multiple fields of study together with industry experts and people from around the world to discuss the latest news in Mars exploration, as well as what is needed so humans can eventually live on Mars.
In the course of attending the conference, Morgan and Lee became concerned that the focus of this and other conferences like it was still on the history of Mars exploration and the technology that will be needed to make the journey. What was missing was the question of what humans will do once they get to Mars; namely, how will they grow their own food in a way that is sustainable? As Lee recounted:
“When I saw that young people were still being encouraged to apply to be an astronaut if they desired to work in the space industry, I knew that the space industry as it had existed was not going to get the job done. And we are not he only ones who saw this. We know many who started their New Space businesses around the same time.”
While plenty of research has been aimed at determining if Earth crops can grow in Martian regolith, virtually nothing has been done to address how that soil could be kept rich in organic molecules and minerals (i.e. fertile) over time. Since she was on the cusp of completing a double honors thesis in Environmental Science and Biology at Duke University, North Carolina, Morgan was both fascinated and eminently qualified to tackle this question.
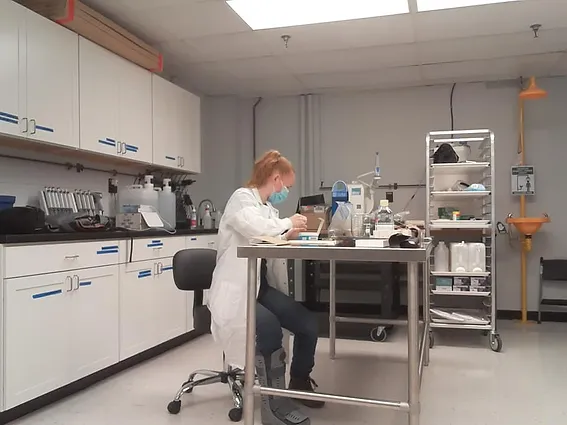
Since January of 2015, Morgan had been researching a Closed-Ecological System (CES) model that would enable sustainable space habitation. In the school year leading up to her trip to the H2M conference, she developed an experimental procedure for testing pretreatments in Martian regolith to help plants grow in it. While she was at the conference, the Duke University Greenhouse was receiving her shipment of Mars regolith simulant.
The fruits of this work include a patented CES model that utilizes three zones to ensure sustainable agricultural practices and food security for off-world habitats. The three zones, in their simple arrangement, form concentric circles and consist of an ecological buffer zone (outer circle), an agricultural zone (second circle), and human habitation (innermost circle).
According to the patent (filed provisionally in July of 2016 and granted in May of 2018), this model “mimics the self-sustaining and resilient capacity of Earth” and would allow for the creation of “self-sustaining and resilient human habitation on the Moon and Mars and for food security and climate change mitigation anywhere on Earth.”
Currently, Morgan is working towards her Ph.D. at the Lehmann Lab at Cornell University, where she is studying bacterial organic adhesion mechanisms in soil aggregates and their effects on soil organic carbon persistence. Her ultimate research interest is to understand how biogeochemical cycles are established in soils here on Earth and on other astronomical bodies.
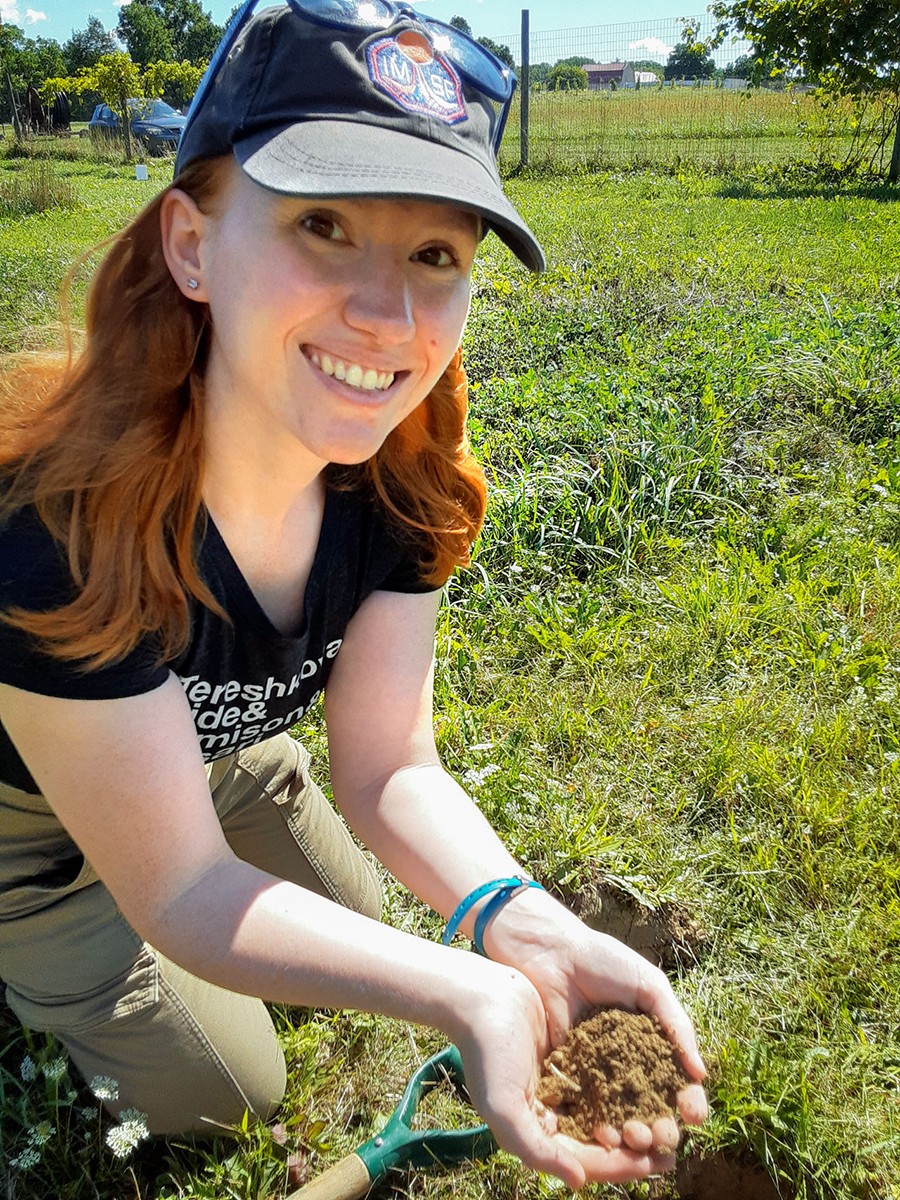
The Soil Health in Space experiment is part of her Ph.D. research, which is largely focused on determining what role (if any) gravity plays in microbial-driven soil aggregation and how this affects overall soil health. The results of this experiment will apply to future space missions, but also here at home, where soil degradation is happening due to unsustainable, intensive agriculture and climate change.
Morgan is also a 2020 National Science Foundation (NSF) Graduate Research Fellow, a 2019 Norfolk Institute Fellow, and the recipient of the 2019 Ken Souza Memorial Spaceflight Award from the American Society for Gravitational and Space Research (ASGSR). Her father, Lee Irons, is also a scientist, as well as an engineer and entrepreneur.
His previous work includes research into space plasma physics, energy production, the decontamination and remediation of hazardous environments, and large-scale engineering and construction projects – all of which he has parlayed into creating solutions for off-world living.
This same purpose motivated Lee and Dan Lopez (entrepreneur, technologist, and a member of the SETI Institute‘s Council of Advisors) to create the Norfolk Institute. Like DSE, this non-profit organization is dedicated to creating food security systems that will ensure human resiliency in space and on Earth. Said Lee:
“There is a certain amount of research and development that is best accomplished under non-profit means by for-profit interests to help whole industries. We started Norfolk Institute to do this type of work in support of the missions of companies such as Deep Space Ecology that are trying to help the human species survive and thrive on our Earth and in our universe.“
Gravity and Soil
Far from being dead or inert, soils are living, breathing entities that are filled with microorganisms, soil fauna, nutrients, organic matter, and minerals. Its ability to form clumps (aggregates) is central to ensuring that it sustains its structure and quality over time since aggregates play a big role in regulating the important biogeochemical processes that make soil fertile. As Morgan Irons explained to Universe Today via Zoom:
“Aggregates are part of the soil structure, and depending on the size of aggregate, it will affect the porosity of your soil. Porosity is, just as it sounds, the pores that are in your soil structure. Pores are so important because they allow for water to infiltrate down into the soil column, [they] allow air to come in and be exchanged and they also allow plant roots to easily penetrate. Aggregates create this porosity structure in your soil which allows these things to happen.“
But, as Morgan added, the role of aggregates go beyond merely helping maintain soil structure. According to multiple lines of evidence, it is theorized that they protect organic matter over the long-term as well. This is due to the fact that not all of the organic matter that’s added to soil is automatically broken down by microorganisms into its chemical constituents that are immediately consumed by plants or leached down into the soil columns.
In some cases, organic molecules will have soil minerals occlude to it, creating a protective envelop that the bacteria cannot penetrate. Said Morgan:
“Organic matter can be protected within these soil aggregates, and this leads to long-term sequestration or long-term persistence of soil organic matter. Soil organic matter or organic carbon persistence is highly important for the fertility of the soil because you don’t want your nutrient source to just run right through the soil column or get decomposed quickly. If you’re not meeting the rate of input to the rate of uptake, your soil is going to be pretty nutrient-deficient. So having organic matter that is sequestered away can help with that long-term fertility of your soil.”

The purpose of their Soil Health in Space experiment is to see what (if any) effect microgravity will have on a soil sample’s ability to form micro-aggregates or macro-aggregates that will sequester nutrients. Macro-aggregates are large clumps of dirt that one would find if they dug their hands into a pile of soil, micro-aggregates are much finer.
Since soil comes in many different varieties, which has a bearing on their ability to grow plants, Morgan and her colleagues chose three soil samples for their experiments. These samples fall into three distinct categories: fibrous, organic, and clay-rich (silty), and were chosen based on their particular compositions and textures.
The fibrous sample was provided by Bio365, a commercial manufacturer of nutrient-dense soils for commercial agriculture that is based in Ithaca, NY. This soil was specifically engineered and fortified with biochar (natural charcoal produced from decomposed biomass) to ensure that it was loaded with nutrients and organic material.
The organic sample, meanwhile, is a mineral soil provided by Dr. Matthias Rillig (a professor of biology) at Freie University Berlin. Composed mainly of concentrated sand, this soil is highly porous and made up of larger sand particles. It also contains lots of organic material and has a high density, which makes it “hydrophobic (meaning that it doesn’t absorb water well).
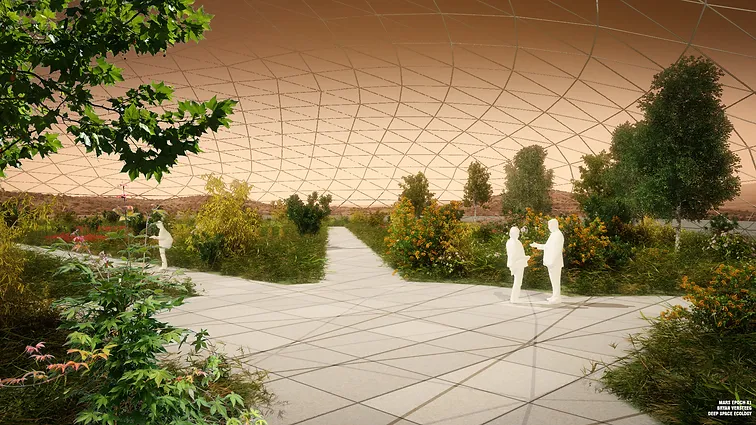
The clay-rich sample also came from Ithaca, specifically from an organic farm on the campus of Cornell University. This type of soil is common in the New York state and dates back to the last glacial period (ca. 115,000 – 11,700 years ago). Its material origins (parent material) are “glaciolacustrine,” because it came from the retreating ice sheets that scraped up material from the Great Lakes region and deposited elsewhere as soil.
Compared to the other samples, this soil has smaller pore sizes and a better chance of aggregating than the sandy soil (the Berlin sample). Between these samples, Morgan is investigating the effects of gravity (or lack thereof) on fungal mycelial production and bacterial organic adhesive (holdfast) production in soil, the resulting effects on soil aggregate stability, and the occlusion of organic carbon within those aggregates.
Understanding gravitational effects on the functional and ecological dynamics of soil microorganisms and thus soil biogeochemistry would allow for farming in space, thus supporting the goals of Deep Space Ecology. As Morgan put it:
“The different textures between these mineral soils allows us to study the potential formation of micro-aggregates vs. macroaggregates, to see if they can actually form in a microgravity environment. And if they form in a microgravity environment, we ask the question, ‘is it because of the microorganisms, producing organics adhesives in the soil that create the necessary organic-mineral interactions?’ If these aggregates are still forming, it likely means that the microbial mechanism that’s allowing this to happen isn’t necessarily gravity-dependent.”
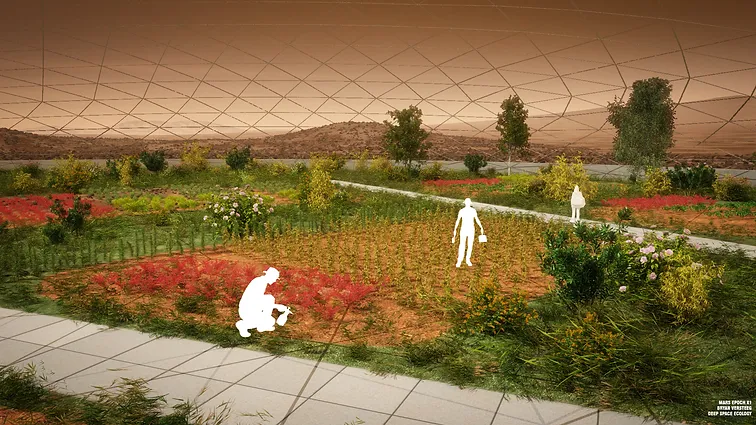
In short, the experiment seeks to investigate whether or not gravity is part of the mechanism that allows microorganisms to perform the function of aggregate formation. Given that these microorganisms evolved in soils here on Earth, it is widely assumed that if they are taken out of Earth’s gravity, they may not function the same. But as Morgan indicated, plant studies aboard the ISS have already shown that this isn’t always the case.
“Sometimes gravity being this huge force vector has this masking effect, where it covers or hides what’s actually happening on that fundamental, mechanistic level,” she said. “So if you take that huge force vector out of that equation, and everything is still functioning the same, you have to ask ‘what’s actually driving this plant, this system, to work this way.'”
The Experiment
The Soil Health In Space experiment consists of the three soil samples each being divided up and placed into 12 vials of 4 ml (0.135 fluid oz). Each set of 12 was then subdivided into two groups of six, which Morgan calls the “free-floating” and “restricted movement” group. In the former, the soil is able to float freely in microgravity, while the latter was equipped with mesh stoppers that hold the soil down but allow water and air to get through.
As Morgan said, the purpose of this is to see what effect being allowed to free-float vs being held close together will have on the soil’s microbiome. And they anticipate that the results will be a bit mixed:
“Allowing free-floating vs. restricted movement allows us to test the abiotic effect of soil structure on the soil microbiome. This gets into one of our hypotheses where we think the fungi aren’t necessarily going to do as well if the soil is allowed to float freely. So we might see a shift in the population where the fungi populations might decrease while the bacteria populations either stay the same or increase.
“Bacteria tend to say where they are – they adhere to a mineral, they adhere to organic matter, and they are good to go. While with fungi, they rely on their network of mycelium across the soil sample. And if you break that structure up, where it’s just free-floating now, the fungi might not do as well. This will, of course, affect the abundance and stability of the aggregates we may or may not find in the samples.”
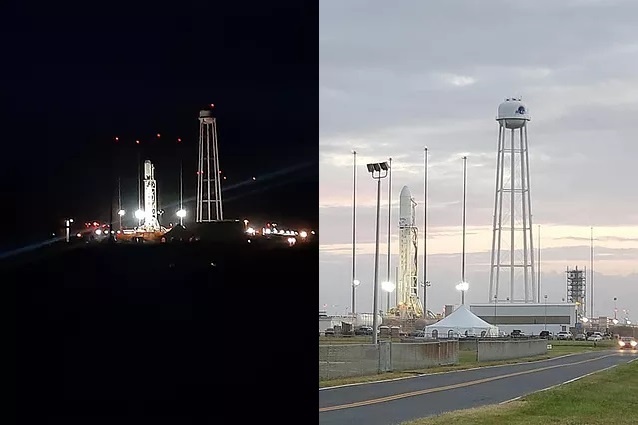
But it doesn’t end there. For the next experiment treatment, the restricted movement and free-floating samples were each subdivided into two groups of three, which were then administered water at 60% and 30% of their respective holding capacities. As Morgan explained, all the samples were had to be air-dried beforehand and could only be watered at the launch site (which she did herself_)shortly before blastoff:
“Right before launch, right before handing them off, I [gave them] water to activate them. Because as soon as water hits them and is allowed to get into that environment, the microorganisms start amping up their microbial processes. Using 30% vs. 60% water holding capacity allows us to test the biotic effect of microbial activity on the soil – 30% is drought-like conditions while 60% is optimal water conditions. Microorganisms in the 30% tubes might not perform to the same capacity as the microorganisms in the 60% tubes.”
As of Oct. 15, NASA indicated via the ISS On-Orbit status Report that the crew had removed the experiment’s five chambers from their stow location aboard the Cygnus spacecraft and placed the samples into cold storage.
Solving for Earth
To quote famed science communicator, Dr. Sian Proctor, “Solving for space solves for Earth.” This is especially true when describing Deep Space Ecology and their experiments, which are focused on developing systems that leverage modern science and biology with ancient knowledge and “best practices” that will allow humans to farm in extreme environments.
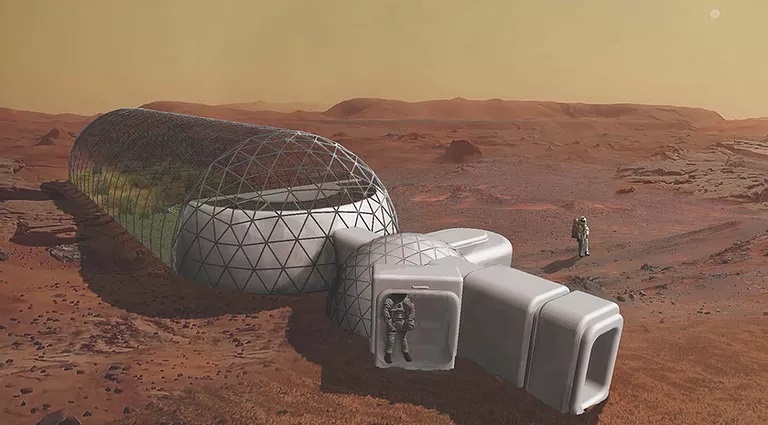
After all, agriculture is the oldest science known to humanity, one that has existed for over 11,500 years. And as Morgan put it, our ancient ancestors who practiced it were scientists themselves – after a fashion:
“For thousands of years, we’ve had indigenous groups of people practicing agriculture and they’ve been able to do so sustainably because they’ve [learned] through trial and error. They themselves [were] scientists by learning what worked, what didn’t work, they understood how to work with their environment in a sustainable way that took into account how ecosystems function and how you can maintain it for generations upon generations.”
A good example, which Morgan cites, is the Nabatean civilization that lived in the Negev desert – between modern-day Jordan, Egypt, and Saudi Arabia – from the 4th century BCE to 106 CE, centered around the ancient city of Petra. At its height, this civilization was able to support a population of 30,000 people in the desert thanks to their system of agriculture and water transport.
The feats of engineering that led to this, according to Morgan, continue to astound archaeologists to this day:
“When modern-day scientists and archaeologists started to investigate this area and investigate the city of Petra… what they found was the water collection system, the water storage cisterns, [and] the irrigation systems of piping technology that they used during the 4th century BCE to 106 CE were all ‘best practice.’
“In other words, engineers couldn’t do it any better like if they tried to recreate it in the modern-day with the same materials and it’s because the Nabateans sought to understand their landscape [and] how water naturally moved through their landscape to develop the most efficient and sustainable technology.“
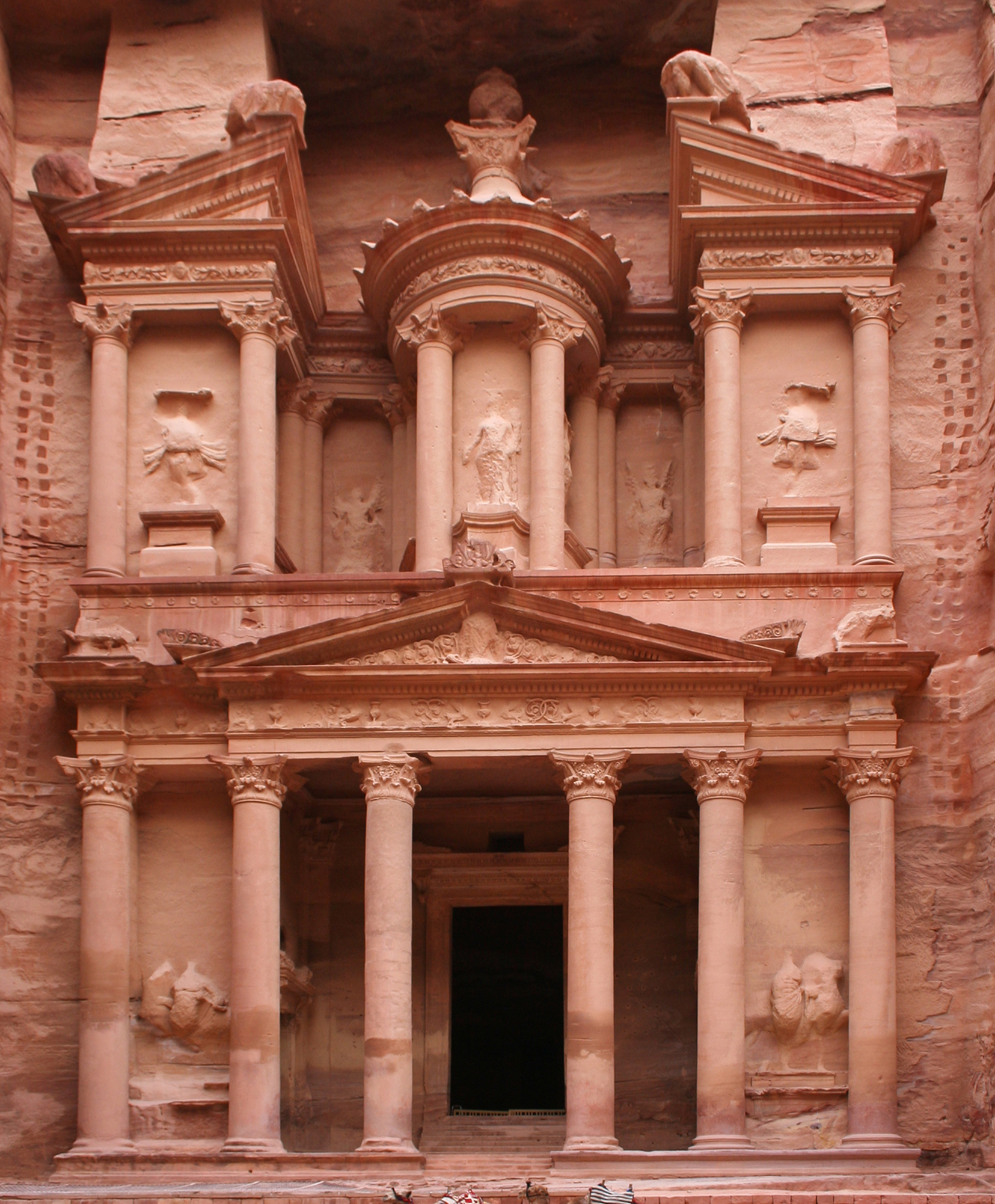
Today, agriculture is much different, owing to a combination of factors. On the one hand, European colonization of the Americas, Africa, and Asia (16th to early 20th century) led to the destruction and forcible removal of indigenous communities. It also led to knowledge and local practices that were all about living sustainably with the land being replaced with European farming practices (which emphasized yield).
Another major factor was the Industrial Revolution (ca. mid-18th to the early 20th century) which resulted in the introduction of farming machinery that could support more large-scale operations. This trend has intensified with the “Green Revolution” of the 1950s and 1960s, which introduced more in the way of chemical fertilizers, insecticides, and herbicides, and intensive land management, as well as the introduction of genetically-modified crops.
These practices are not sustainable and are leading to soil degradation and the disappearance of arable land all over the world. This is happening at a time when the global population continues to expand and more and more land is being set aside for urban infrastructure. It’s also contributing to the growing problem of climate change. As Morgan summarized, soil degradation means less carbon is removed from our atmosphere over time:
“Our terrestrial landscape is the largest sink of organic carbon on Earth. The soil is holding a lot of that carbon in organic forms and so having a soil structure that allows that organic matter to be protected, to persist or be sequestered down in a pool and not be decomposed and released as CO2 back into the atmosphere, is very important for climate change as well.“

In short, developing methods for sustainable agriculture will not only ensure that people travelling to space or living on other planets will have enough to eat, it will also allow for soil rejuvenation here at home. One of the first steps is to determine whether or not soil health and Earth plants need gravity in order to grow and thrive.
The answer to this question will have drastic implications for food production in space, on the Moon, on Mars, and anywhere else in the Solar System humanity chooses to establish a lasting presence. Morgan and her colleagues suspect that the presence of Earth gravity (1 g) may not always be an essential factor, which means that plants could be grown in space or on other terrestrial bodies using real soil (and not just hydroponics).
If, however, Earth gravity is essential, that means that all future space facilities will have to rely on artificial gravity to grow their food. As for places like the Moon and Mars, where the gravity is lower, further experiments will be needed to determine to what extent lower gravity will affect our ability to grow crops.
At present, DSE has produced concepts for three generations of the Mars Quasi-Closed Agro-Ecological System – aka. Mars Epoch X1 to X3 – that would allow for gradual adaptation and settlement on Mars. As you can see from the images posted above (and the video below), these designs call for the creation of closed-ecological systems within sealed and transparent domes, and come in many shapes and sizes.
On the business end of things, Lee Irons indicated that in 2021, DSE will be rolling out a customer interface, where customers can answer specific questions about what they are looking for. This will be paired with a catalog of products and services that DSE will be offering potential customers. This interface will include a soon-to-be-announced Earth line of products and services.
“We have demonstrated that our thinking is in the right direction,” said Lee. “Our Chief Science Officer, Morgan Irons, is a patent holder, a National Science Foundation Graduate Research Fellow and PhD student at the top agricultural university in the world, Cornell University, and currently has an experiment on the International Space Station. We have Earth market solutions and related initiatives planned for which we are seeking investment funding.”
For more information about their research, services, and long-term vision, check out the Deep Space Ecology and Norfolk Institute websites.
Further Reading: Deep Space Ecology, Norfolk Institute