Do you have a few minutes to spare and a thirst for knowledge about one of the greater mysteries of the Universe? Then head on over to ArsTechnica and check out the new series they’re releasing titled Edge of Knowledge, starring none other than Dr. Paul Sutter. In what promises to be an enlightening journey, Dr. Sutter will guide viewers through an eight-episode miniseries that explores the mysteries of the cosmos, such as black holes, the future of climate change, the origins of life, and (for their premiere episode) Dark Matter!
As far as astrophysicists and cosmologists are concerned, Dark Matter is one of the most enduring, frustrating, and confusing mysteries ever! Then, one must wonder why scientists are working so tirelessly to track it down? The short answer is: the most widely accepted theories of the Universe don’t make sense without out. The long answer is… it’s both complicated and long! Luckily, Dr. Sutter manages to sum it all up in less than 15 minutes. As an accomplished physicist, he also explains why it is so important that we track Dark Matter down!
Put simply, Dark Matter is a perfect place to start a series that deals with the “Edge of Knowledge” because it is just beyond the edge of scientific knowledge. Thanks to Einstein’s Theory of General Relativity, scientists can calculate how massive objects behave on the largest cosmic scales. However, throughout the 1950s and 60s (the “Golden Age of Relativity”), astrophysicists noticed that the gravitational behaviors they were observing did not conform to the amount of “luminous” (aka. visible) matter.
How Do We Know it Exists?
This part of Dr. Sutter’s explanation takes the cake! Like Galileo and Einstein before him, he illustrates how scientists study Dark Matter using a simple metaphor: a person standing on a ship at sea. “[I]t’s the middle of the night and you see lights on a distant shore,” he says. “Now, those lights tell you that the shore exists, but it doesn’t tell you anything about it. There could be mountains or jungles, you just don’t know. This is the situation with dark matter.”
The evidence for it is all around us. As Dr. Sutter said cheekily, “I mean, I wrote a book about it!” From a copy he happened to have on hand, he lists the lines of evidence. “There’s the rotation curves of galaxies. There’s the temperatures of galaxy clusters. There’s the bending of light around massive structures. There’s the large-scale structure of the Universe itself. There’s the cosmic microwave background. It goes on and on and on and on.”
Cold, Collisionless, and Abundant
But what do we actually know about it? Well, for starters, we know what it isn’t, which has allowed theoretical astrophysicists to place constraints on what it might be. As Dr. Sutter explains, what we have learned about Dark Matter (DM) states that it conforms to three parameters: Cold, Collisionless, and Abundant. This forms the basis for the most widely accepted model of the Universe, which is the Lamba Cold Dark Matter (LCDM) model. As Dr. Sutton put it:
“Whatever dark matter is, it must be cold, collision-less, and abundant. We’d like to know what dark matter is made of because it can help us understand the dynamics of galaxies and the evolution of the universe. Oh, and by the way, it is by far the most common particle in the universe. So, it’d be nice to know what it’s actually made of.”
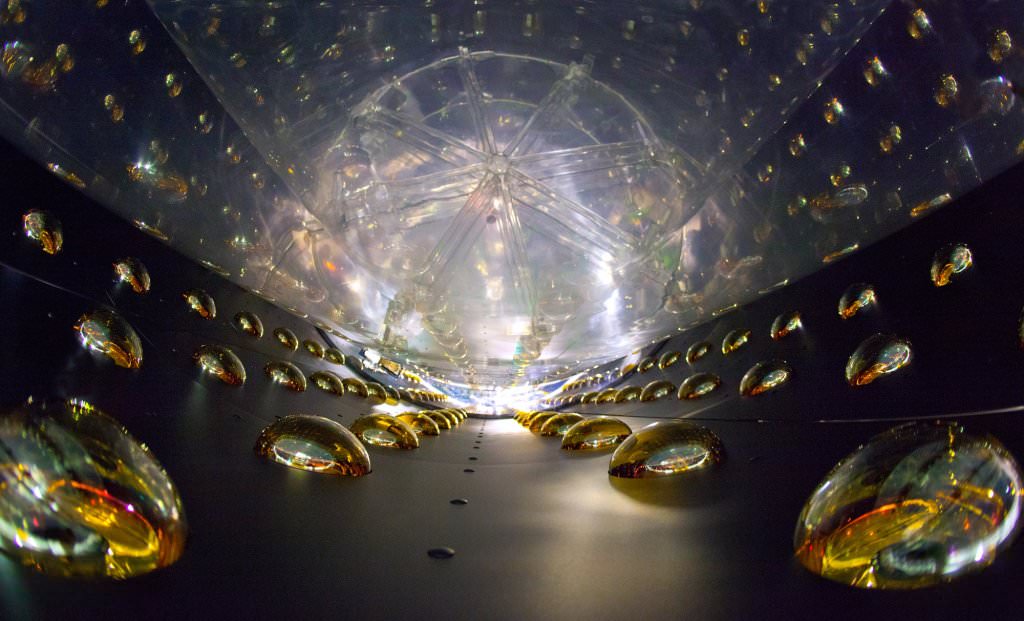
This is one of the episode’s high points, where Dr. Sutton provides an insightful yet straightforward summary of DM’s theoretical and experimental basis (with the assistance of a chalkboard). For the former, he explains how the rotational velocities of galaxies (a result of their mass distribution) show how luminous matter alone cannot account for what we see. There’s also the presence of “Dark Matter Halos,” which every galaxy in the Universe appears to have.
“This scale density and scale radius are different numbers for every single halo, but no matter what, they all share this common shape and the shape looks like this, density as a function of radius, starts like this and then goes down,” he said. “And the scale radius tells us where this breaking point is. It tells us something interesting about the evolution of dark matter halos.”
In essence, says Dr. Sutter, these Halos suggest DM forms as a dense, centralized, ball-like structure that accumulates more DM particles over time. The formation of these halos is intrinsically linked to the formation and evolution of galaxies and the large-scale structure of the Universe. This is one of the many reasons scientists are so excited about the James Webb, Nancy Grace Roman, Euclid, and other missions that will observe how the Universe evolved from the beginning – it would be a chance to see this process at work!
Candidate Particles
As for detecting what DM is made of, Dr. Sutter provides a rundown with the assistance of an aquarium, lots of plastic balls (6m50s into the video), and a guest expert. As he put it, “every single theorist with time to kill makes up their own pet theory of how dark matter might work, and we have so many candidates.” Examples include WIMPs, self-interacting dark matter, axions, and axion-like particles. We have primordial black holes and sterile neutrinos and on and on and on.
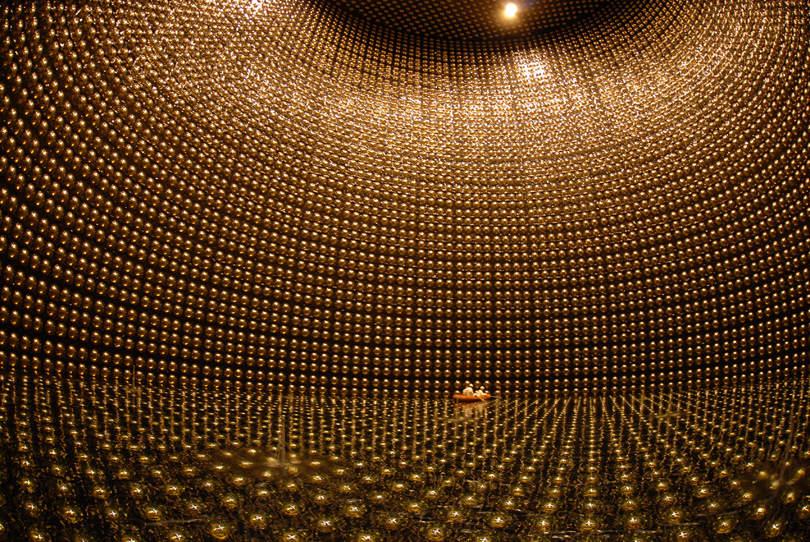
“But what really matters is that all these different ideas, all these different theories predict how dark matter might behave in our universe,” said Dr. Sutter. “And then we can go out and try to detect it, observe it, somehow catch a glimpse of dark matter and prove one of these hypotheses right.” Existing efforts to detect DM candidate particles include neutrino detectors like the IceCube Observatory in Antarctica, the SNOLAB facility in Ontario, and the Super-Kamiokande Observatory in Japan (picture above).
These facilities are usually located underground and operate in total darkness to ensure no background interference from EM sources. This way, the detectors can spot the tiniest releases of energy that may result from interaction between DM candidate particles or a one-in-a-million chance of interaction with normal matter. To do justice to this aspect of DM, Dr. Sutter calls upon famed theoretical cosmologist Prof. Janna Levin – the Claire Tow Professor of Physics and Astronomy at the Barnard College of Columbia University – to explain further.
A salient point made by Prof. Levin is that DM does exist and has been observed, but that it takes several forms (the bulk of which are not accounted for):
“[N]eutrinos are absolutely a physical undeniable verifiable example of dark matter. They do not interact with light. They have all the properties of dark matter, but they’re not heavy enough or abundant enough to explain the extreme dominance in the energy pie. So, if you think of the energy pie of the universe, dark matter is taking up like some twenty-five percent, let’s just say roughly.
“So, I think the question is really, are there really heavy neutrinos? And that’s basically a lot of people are looking for that. They’re looking for WIMPs… that are much, much heavier than neutrinos and that don’t fit into our standard understanding of particle physics.”

Another important point she addresses is that there are many unresolved questions concerning DM’s involvement in the evolution of the Universe. This goes beyond the evolution of galaxies and includes questions about “baryogenesis” and matter/anti-matter asymmetry. These refer to the birth of baryonic (normal) matter in the Universe and how matter must have exceeded anti-matter in the earlUniversese (respectively).
“We know that for some reason, matter is preferred over anti-matter,” said Prof. Levin. “And so there’s a tiny, tiny excess. Should dark matter play a role in that? Probably, one would hope so by the economy of explanations, but we don’t really know. So, if we find the dark matter, for sure the hope is that we’re going to be like, ‘Whoa, does it explain baryogenesis and where does it fit into the bigger scheme?'”
Yes, it’s a big, frustrating, and complex matter. But after listening to Dr. Sutter and Prof. Levin go through all of the pertinent details, it becomes clear why DM and the search for it are so important to modern science. As Dr. Sutter summarizes, “Dark matter is more than a hypothesis. It’s a framework for understanding vast swaths of phenomena across the Universe, but it’s like a house that isn’t finished. We have the foundation. We just can’t live in it yet. And that’s why dark matter is on the edge of knowledge.”
Stay tuned for future episodes, which are likely to tackle matters of equal importance and complexity!
In addition to being a Research Professor at Institute for Advanced Computational Science (IACS) at Stony Brook University and a Guest Researcher at the Flatiron Institute, Dr. Sutter is also a renowned author and a regular contributor to Universe Today! If you’ve got time after the video, check out his extensive catalog of articles, especially his most-recent series that explains all the complex terminology in astronomy: Astronomy Jargon 101.
Further Reading: ArsTechnica
It’s not bad, since Paul Sutter isn’t as frantic as his ordinary oeuvre, and of course the physics is sound.
Just a note here, I suspect that Levin was referring to sterile neutrinos which are ordinary “massive” neutrinos heavier non-weakly interacting relatives if they exist. Supersymmetry neutralinos is something else and supersymmetry has refused to turn up in the LHC as it should.