According to the most widely accepted cosmological theories, the first stars in the Universe formed a few hundred million years after the Big Bang. Unfortunately, astronomers have been unable to “see” them since their emergence coincided during the cosmological period known as the “Dark Ages.” During this period, which ended about 13 billion years ago, clouds of gas filled the Universe that obscured visible and infrared light.
However, astronomers have learned that light from this era can be detected as faint radio signals. It’s for this reason that radio telescopes like the Murchison Widefield Array (MWA) were built. Using data obtained by this array last year, an international team of researchers is scouring the most precise radio data to date from the early Universe in an attempt to see exactly when the cosmic “Dark Ages” ended.
As they indicated in their study, which appeared in The Astrophysical Journal last year, the team had succeeded in filtering out ten times as much electromagnetic interference (compared to the norm) from more than 21 hours of data collected by the MWA. Equipped with this vastly-improved data, the team is now searching through it all for telltale indicators of a radio signal that originated from the “Dark Ages.”
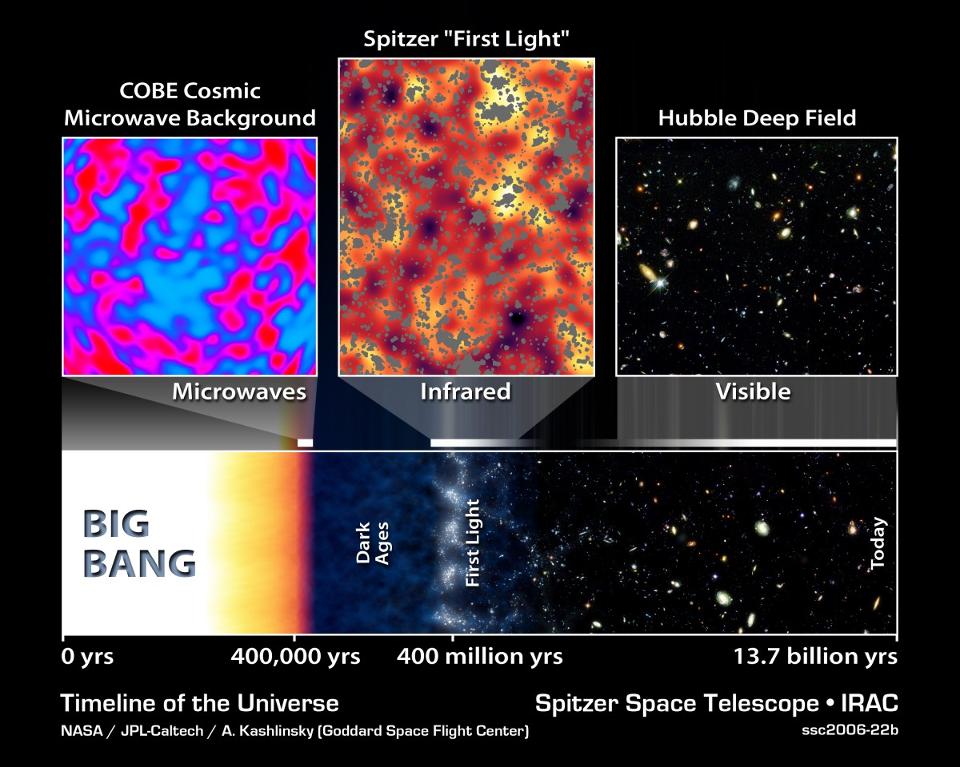
Current models of cosmology tell us that shortly after the Big Bang, the Universe was filled with hot dense plasma. Electrons and photons regularly snared each other in this period, which made the Universe opaque. Less than a million years later, electron-photon interactions became rare and the expanding Universe became increasingly transparent. However, there were still no stars or galaxies at this time, which left the Universe dark.
This is how things would remain for the next few hundreds of millions of years, where the cosmos was filled with neutral hydrogen (hydrogen atoms with one proton and one electron, which have has no overall charge). By about 1 billion years after the Big Bang, neutral hydrogen atoms began to come together to form the first stars, thus beginning the Epoch of Reionization and ending the “Dark Ages.”
As the first stars formed, the light and radiation they emitted converted much of the neutral hydrogen in the Universe into ionized plasma, which still dominates interstellar space today. Unfortunately, discerning when this transition occurred is difficult since electromagnetic radiation in the visible and infrared (heat) wavelenghts are simply not visible where existing instruments are concerned.
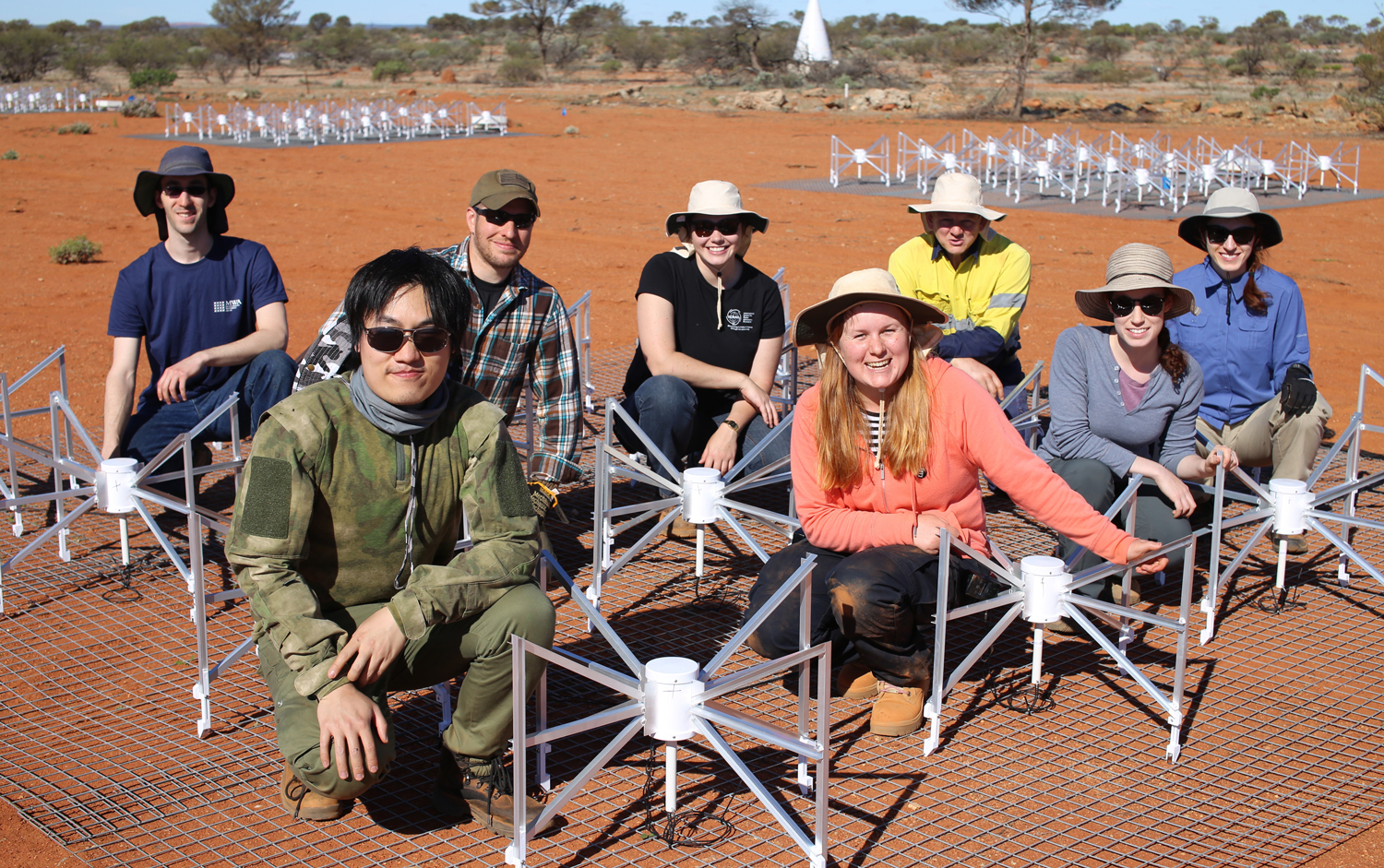
As Miguel Morales, a UW professor of physics and the lead author on the paper, explained in a UW News release:
“For this dark age, of course there’s no light-based signal we can study to learn about it – there was no visible light! But there is a specific signal we can look for. It comes from all that neutral hydrogen. We’ve never measured this signal, but we know it’s out there. And it’s difficult to detect because in the 13 billion years since that signal was emanated, our universe has become a very busy place, filled with other activity from stars, galaxies and even our technology that drown out the signal from the neutral hydrogen.”
This signal corresponds to the frequency and wavelength at which hydrogen goes from being neutral to carrying a charge. Also known as the “Hydrogen Line,” this boundary is found at 21 cm (1,420.4 MHz) on the radio spectrum. Finding these signals in space is the key to determining when the first stars formed and when the Dark Ages ended, thus providing astronomers with vital clues about cosmic evolution.
“The Epoch of Reionization and the dark age preceding it are critical periods for understanding features of our universe, such as why we have some regions filled with galaxies and others relatively empty, the distribution of matter and potentially even dark matter and dark energy,” Morales added.
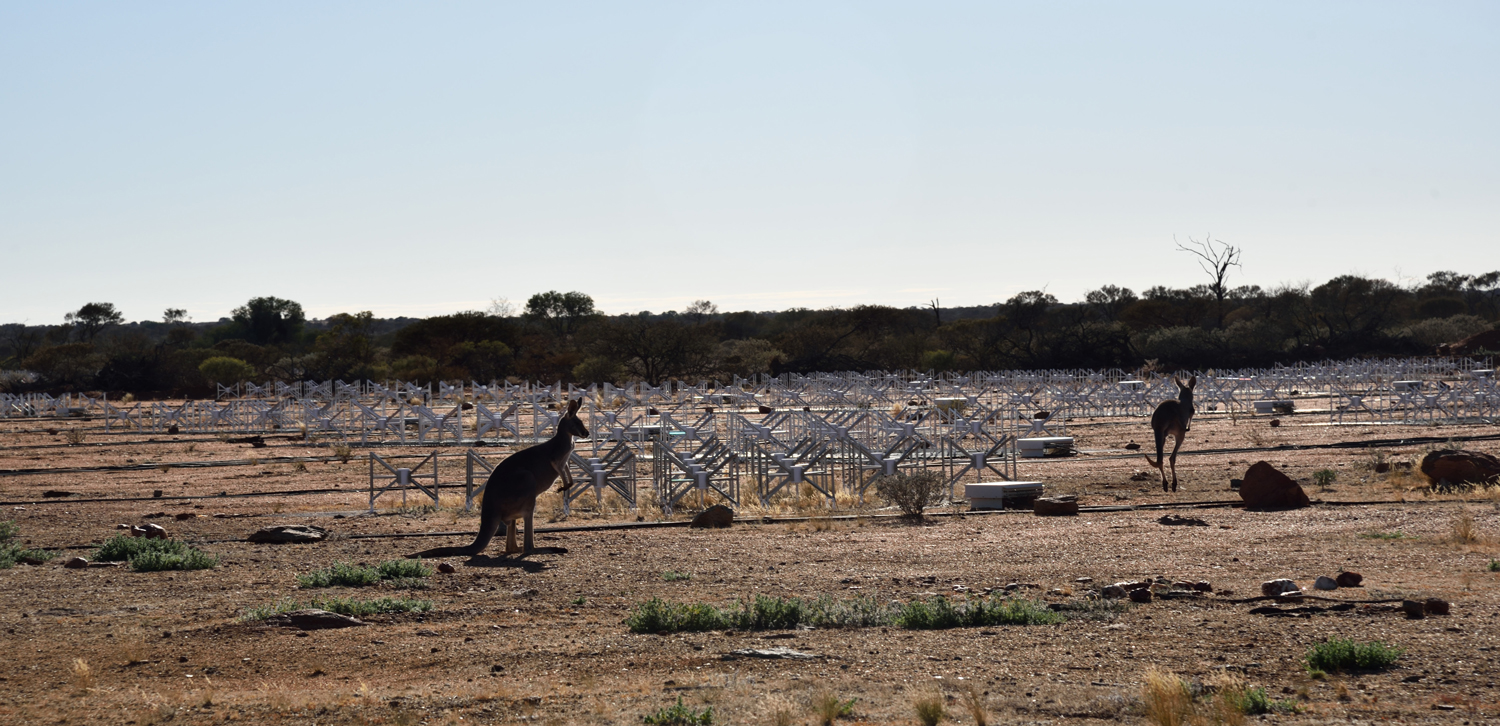
The Murchison Widefield Array, a radio telescope located in Western Australia, is the team’s primary too in searching for these signals. This array consists of 4,096 dipole antennas that can pick up low-frequency signals like the electromagnetic signature of neutral hydrogen. These signals are very hard to discern due to electromagnetic interference from other cosmic (or Earth-bound) sources.
In order to eliminate this background noise and improve the odds of detection, Morales and his colleagues have developed increasingly sophisticated methods. This includes the introduction of a redundant subarray of hexagonal cores and pseudo-random baselines, as well as improved analysis techniques, data quality control methods, and interferometric calibration approaches.
All of this information was present in the team’s 2019 study, which was based on the doctoral thesis of team-member and co-author Nichole Barry – currently a postdoctoral researcher at the University of Melbourne. Looking ahead, the team plans to sort through about 3,000 hours of additional emission data collected by the MWA, and hope to filter out even more noise so they can spot that elusive signal.
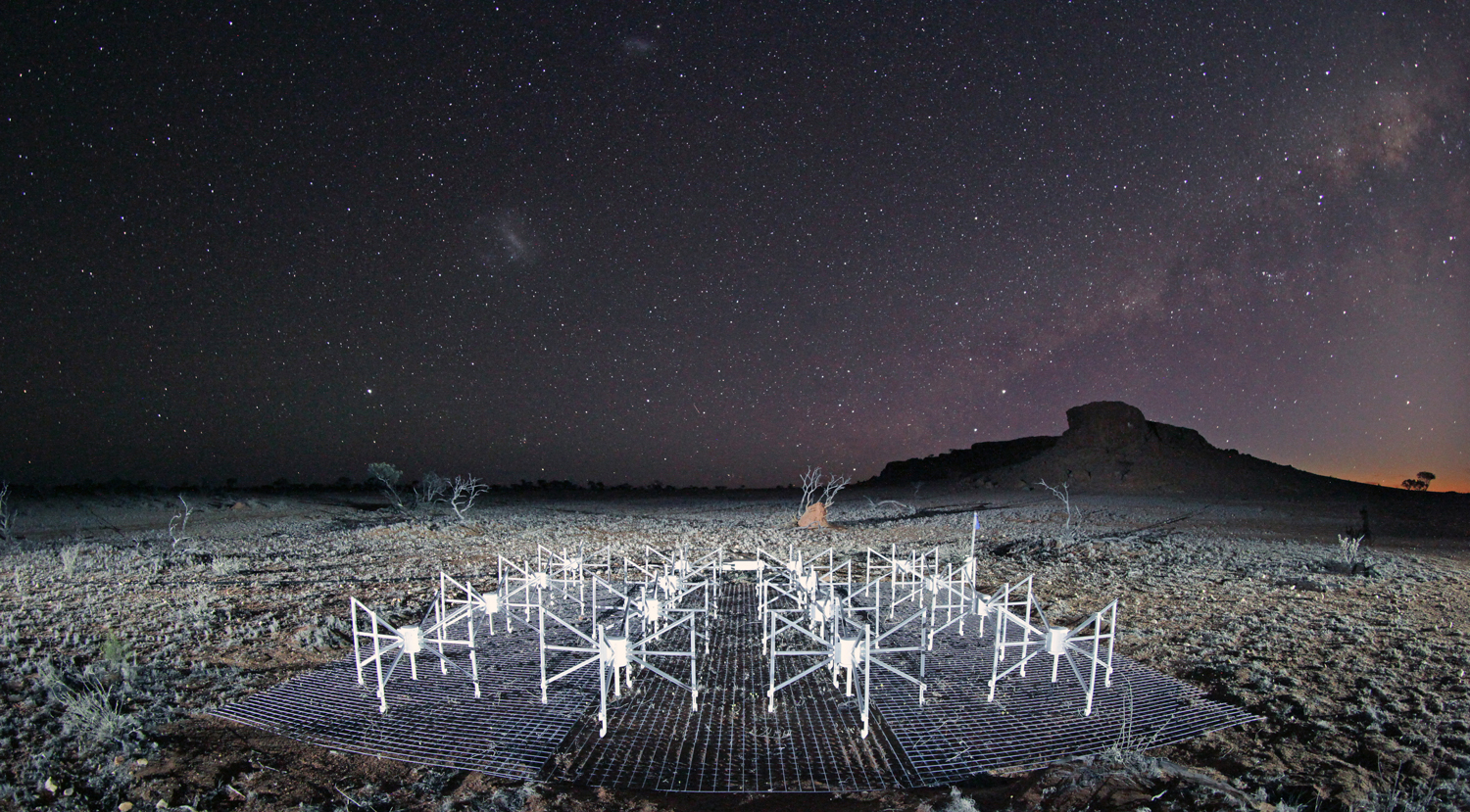
If they are successful, astronomers will finally have a window into the earliest period of the Universe. Along with radio telescope arrays around the world, which are becoming increasingly sophisticated, this will allow astronomers and cosmologists to test their theories about how and when major events in the evolution of our Universe took place. Said Morales:
“We think the properties of the universe during this era had a major effect on the formation of the first stars and set in motion the structural features of the universe today. The way matter was distributed in the universe during that era likely shaped how galaxies and galactic clusters are distributed today.”
The team included researchers from the ASTRO 3D collaboration, the International Centre for Radio Astronomy Research (ICRAR), the Netherlands Institute for Radio Astronomy (ASTRON), the CSIRO Astronomy and Space Science (CASS), MIT, the Shanghai Astronomical Observatory, the School of Earth and Space Exploration, Arizona State University, Kumamoto University in Japan, and Raman University in India.
Further Reading: UW News, The Astrophysical Journal