As we’ve reported recently, the likelihood of findings habitable Earth-sized worlds just seems to keep getting better and better. But now the latest calculations from a new paper out this week are almost mind-bending. Using what the authors call a “very careful extrapolation” of the rate of small planets observed around M dwarf stars by the Kepler spacecraft, they estimate there could be upwards of 100 billion Earth-sized worlds in the habitable zones of M dwarf or red dwarf stars in our galaxy. And since the population of these stars themselves are estimated to be around 100 billion in the Milky Way, that’s – on average – an Earth-sized world for every red dwarf star in our galaxy.
Whoa.
And since our solar system is surrounded by red dwarfs – very cool, very dim stars not visible to the naked eye (less than a thousandth the brightness of the Sun) — these worlds could be close by, perhaps as close as 7 light-years away.
With the help of astronomer Darin Ragozzine, a postdoctoral associate at the University of Florida who works with the Kepler mission (see our Hangout interview with him last year), let’s take a look back at the recent findings that brought about this latest stunning projection.
Back in February, we reported on the findings from Courtney Dressing and Dave Charbonneau from the Center for Astrophysics that said about 6% of red dwarf stars could host Earth-size habitable planets. But since then, Dressing and Charbonneau realized they had a bug in their code and they have revised the frequency to 15%, not 6%. That more than doubles the estimates.
Then, just this week we reported how Ravi Kopparapu from at Penn State University and the Virtual Planetary Lab at University of Washington suggested that the habitable zone around planets should be redefined, based on new, more precise data that puts the habitable zones farther away from the stars than previously thought. Applying the new habitable zone to red dwarfs pushes the fraction of red dwarfs having habitable planets closer to 50%.
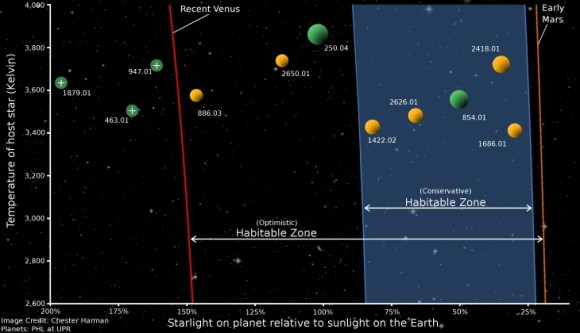
But now, the new paper submitted to arXiv this week, “The Radius Distribution of Small Planets Around Cool Stars” by Tim Morton and Jonathan Swift (a grad student and postdoc from Caltech’s ExoLab) finds there is an additional correction to the numbers by Dressing and Charbonneau numbers.
“This is basically due to the fact that there are more small planets than we thought because Kepler isn’t yet sensitive to a large number that take longer to orbit,” Ragozzine told Universe Today. “Accounting for this effect and enhancing the calculation using some nice new statistical techniques, they estimate that the Dressing and Charbonneau numbers are actually too small by a factor of 2. This puts the number at 30% in the old habitable zone, and now up to about 100% in the new habitable zone.”
Now, it is important to point out a few things about this.
As Morton noted in an email to Universe Today, it’s important to realize that this is not yet a direct measurement of the habitable zone rate, “but it is what I would classify as a very careful extrapolation of the rate of small planets we have observed at shorter periods around M dwarfs.”
And as Ragozzine and Morton confirmed for us, all of these numbers are based on Kepler results only, and so far, while there confirmed planets around M dwarfs, there are none confirmed so far in the habitable zone.
“They do not use any results from Radial Velocity (HARPS, etc.),” Ragozzine said. “As such, these are all candidates and not planets. That is, the numbers are based on an assumption that most/all of the Kepler candidates are true planets. There are varying opinions about what the false positive rate would be, especially for this particular subset of stars, but there’s no question that the numbers may go down because some of these candidates turn out to be something else other than HZ Earth-size planets.”
Other caveats need to be considered, as well.
“Everyone needs to be careful about what “100%” means,” Ragozzine said. “It does not mean that every M dwarf has a HZ Earth-size planet. It means that, on average, there is 1 HZ-Earth size planet for every M dwarf. The difference comes from the fact that these small stars tend to have planets that come in packs of 3-5. If, on average, the number of planets per star is one, and the typical M star has 5 planets, then only 20% of M stars have planetary systems.”
The point is subtle but important. For example, if you want to plan new telescope missions to observe these planets, understanding their distribution is critical, Ragozzine said.
“I’m very interested in understanding what kinds of planetary systems host these planets as this opens a number of interesting scientific questions. Discerning their frequency and distribution are both valuable.”
Additionally, the new definition of the habitable zone from Kopparapu et al. makes a big difference.
As Ragozzine points out:
“This is really starting to point out that the definition of the HZ is based on mostly theoretical arguments that are hard to rigorously justify,” Ragozzine said. “For example, a recent paper came out showing that atmospheric pressure makes a big difference but there’s no way to estimate what the pressure will be on a distant world. (Even in the best cases, we can barely tell that the whole planet isn’t one giant puffy atmosphere.) Work by Kopparapu and others is clearly necessary and, from an astrobiological point of view, we have no choice but to use the best theory and assumptions available. Still, some of us in the field are starting to become really wary of the “H-word” (as Mike Brown calls it), wondering if it is just too speculative. Incidentally, I much, much prefer that these worlds be referred to as potentially habitable, since that’s really what we’re trying to say.”
However, Morten told Universe Today that he feels the biggest difference in their work was the careful extrapolation from short period planets to longer periods. “This is why we get occurrence rates for the smaller planets that are twice as large as Dressing or Kopparapu,” he said via email.
He also thinks the most interesting thing in their paper is not just the overall occurrence rate or the HZ occurrence rate even, but the fact that, for the first time, they’ve identified some interesting structure in the distribution of exoplanet radii.
“For example, we show that it appears that planets of roughly 1 Earth radius are actually the most common size of planet around these cool stars,” Morton said. “This makes some intuitive sense given the rocky bodies in our Solar System—there are two planets about the size of Earth, making it the most common size of small planet in our system too! Also, we find that there are lots and lots of planets around M dwarfs that are just beyond the detection threshold of current ground-based transiting surveys—this means that as more sensitive instruments and surveys are designed, we will just keep finding more and more of these exciting planets!”
But Ragozzine told us that even with all aforementioned caveats, the exciting thing is that the main gist of these new numbers probably won’t change much.
“No one is expecting that the answer will be different by more than a factor of a few – i.e., the true range is almost certainly between 30-300% and very likely between 70-130%,” Ragozzine said. “As the Kepler candidate list improves in quantity (due to new data), purity, and uniformity, the main goal will be to justify these statements and to significantly reduce that range.”
Another fun aspect is that this new work is being done by the young generation of astronomers, grad students and postdocs.
“I’m sure this group and others will continue producing great things… the exciting scientific results are just beginning!” Ragozzine said.
Wow. That is all.
this is massive and there is around 200 billion galaxies in the known universe as well, this is massive beyond all imagination
100 milliards de planetes potentiellement habitables DANS NOTRE Galaxie qui hébergerait Environ 900 MILLES civilisations Intelligentes!
DANS Notre univers combien de galaxies? QUELQUES milliards x 900 milles civilisations. FAITES le calcul? si on rajoute les milliards d’univers (multivers et parallèles) le Chiffre devient ……. infini!
http://alexbabeanu.blogspot.com/2011/11/quelle-realite.html
The inevitable question… What effect do these new findings have for ‘dark matter’ calculations? Sounds like since there’s a whole lot more MACHO’s out there. the numbers need to be revisited?
“Dark matter” isn’t just made up of one thing. It’s a combination of everything we can’t see, or can see but can’t accurately account for.
That said, these planets are utterly immaterial. Their combined mass is so small that it’s irrelevant.
If “baryonic” dark matter existed in large enough quantities to produce the observed results (like the bullet cluster), it wouldn’t be invisible. It would glow enough in the infrared that we’d see it. Therefore, baryonic dark matter on its own cannot explain the observed weirdness. Even adding up every weird source of normal-ish stuff you can think of, like brown dwarfs, rogue planets, wandering black holes, and neutroinos you can’t get anywhere even close to the total amount of mass needed to wipe out the need for more exotic types of dark matter.
It is only “inevitable” if you haven’t familiarized yourself with dark matter and the surveys that shows that no amount of baryonic matter can account for DM.
It is also, IIRC, directly derivable from the WMAP observations – DM is seen to not (to any large degree) be baryonic matter.
Time for a little old fact check and refresher? http://math.ucr.edu/home/baez/physics/Relativity/GR/dark_matter.html
When scientists say habitable, do they mean for whoever lives on those planets, or habitable for human living and breathing?
Where liquid water can exist on the planet’s surface.
Conditions for liquid water. If the planet looks anything like Earth it will have alkaline hydrothermal vents, and that is now believed to suffice for chemical evolution to inevitably result in cells (aka biological evolution) within some 10-100 of thousand years.
To get to complex multicellular life it is also believed that you need an oxygenated atmosphere and oceans.
Photosynthesis can give that.
Basic photosynthesis is not too complex and it seems life got there early. The earlier controversial status of the Isua metamorphic (heat changed) sediments of 3.7 – 3.8 Ga bp [billion years before present] has likely been resolved as fossil deposits:
“The rocks of this formation can be 3.7-3.8 billion years old, and include the oldest known BIFs on Earth. Ultimately, the isotopic signatures in the rocks suggested that oxidation of Fe(II) was most likely the result of anoxygenic photosynthesis (i.e. photosynthesis that doesn’t produce oxygen).”
This makes likely all BIFs signifying fossils, and these fossils may now be the earliest known life. Validating how easy but powerfully environmentally changing photosynthesis is.
However, oxygenating photosynthesis is another history. In principle it is not so complicated as what eventually evolved. You need to exchange other metal ions in the photosensitive compound to manganese. [ http://en.wikipedia.org/wiki/Photosystem_II ]
It is believed that the anoxygenic photosynthesis changed the oxidation state of the oceans sufficiently that manganese eventually became available in the bulk water volume. [Sorry, can’t find the ref right now.]
Then cyanobacteria, which themselves evolved multicellularity (albeit simple such) during the oxygenation of the atmosphere, evolved since oxygen as the name implies is a good end member for energetic reduction-oxidation chemistry. In short order the cyanobacteria. which originated around 2.8 – 2.5 Ga bp, oxygenated the atmosphere to (an initially small excess) between 2.45 – 2.32 Ga bp. [“Evolution of multicellularity with increased diversification of cyanobacteria and the Great Oxidation Event”, Schirrmeister et al, PNAS 2012]
So first you need to have habitability on a roughly Earth sized planet. Then you need to wait ~ 2 billion years for the atmosphere to turn oxygenated.
The rest of the waiting time until you see complex multicellulars is presumedly waiting to get larger volumes of the ocean oxygenated. That is a more messy affair.
– You need to sequester (capture and bind) sulphides, that kept the oxygenation down by making the oceans toxic. Cyanobacteria did that too, by using nitrates in their specialized nitrogen fixating cells. (Multicellular, remember?)
“”We’ve shown here how feedbacks arising from the fact that life uses nitrate as both a nutrient, and in respiration, controlled the interchange between two ocean states. For as long as sulphidic conditions remained frequent, Earth’s oceans were inhospitable towards complex life.”” [ http://www.astrobiology.com/news/viewpr.html?pid=40261 ]
– You need to sequester carbon, too much methane kept the oxygenation down too. There are ideas that the first minutely sized calcified sponges were doing that, by sedimenting carbon away.
So you need to wait another ~ 1 -1.5 billion years for the oceans to turn oxygenated.
So while a habitable planet may turn inhabitated in short order, it will take some 3 – 4 billion years before you can expect large complex multicellular lifeforms evolving.
There is as of yet little statistics on habitable planet ages. The Habitable Exoplanet database have 4 out of 7 planet/candidates as older than 4 Ga, and 1 as younger than 3 Ga.
I wonder if the inhabitants to red dwarf systems rule out bright stars for life, because red dwarves are so common.
And red dwarfs seem to be one of the most stable entities in the known universe. They might think that our yellow dwarf star is too unstable for life, even though yellow dwarf stars are very stable compared to larger stars.
The bigger question is how many of these potentially habitable planets really are habitable? There are many ways that a planet in the habitable zone can turn out to be nothing like Earth. We need spectral data of their atmospheres to help answer this question.
Ok, now just to resolve a small problem, how to get there ….
Why? These planets are immensely valuable for science as they are, helping constrain planet system, planet formation and life formation theories.
Visiting is more tied to other social values, such as economy. Can you afford visiting them, when you can’t afford visiting the Moon?
That seems about right.
The earlier 100 – 200 total billion planets figure was a lower limit. But the surveys of Kepler’s pipeline implied that there are tens of planets per star on average.
And our own system has 25 % Earth-sized planets (2 out of 8), implying there are at 1-3 Earth-sized planets per star on average. Most of those would, like ours, have a fair chance to be parked in the new, extensive habitable zone.
This would be based on best estimates (aka handwaving) and that Kepler’s baseline has a confirmed ~ 90 % accuracy between candidates and planets. So it is good to see other estimates getting to the same neighborhood.
It is alos good to see how the discussion about habitables have moved beyond the dubitable “tidally locked” presumed showstopper into discussing the problems of diverse (very diverse!) planets in the habitable zone of stars. I like the proposed classification of potentially habitable vs validated habitable (say, Earth-massed planet within the HZ of a G star), but it may also be too coarse to be useful.
Heh. The young stars.
Then if we extrapolate about E.T. the Fermi paradoxe become even more relevant.
That’s what I was thinking.
The universe is so young that we don’t even know what long term effect these stable red dwarf systems will have when/if the red dwarfs survive their host galaxies. If some form of humans exist when the sun dies, the red dwarf might be our destiny. The light from larger, more unstable stars lights up the universe. Just as the mountains in the distance tell us that life is more difficult than on flat ground, the bright lights might suggest a place where it is possible to survive, but not preferred. These numbers suggest that our yellow dwarf neighborhood could be the less common environment where most life in the universe originated.
Yellow dwarfs are believed to live for 10 billion years. Compared to what? 100 billion for a red dwarf? A trillion? Statistically, this is like finding at least 10 yellow dwarf stars when you include the time for opportunities for life to get started. Our system is only 5 billion years old. It took half of our star’s life cycle for humans to show up. This new age of red dwarf awareness does so much more than add mere numbers to the habitable systems available for odds calculations. If each red dwarf lives for 100 billion years on average, it seems to me that the odds for life increase 10 fold when compared to a yellow dwarf system.
See the last paragraph of the “Variability” section at
http://en.wikipedia.org/wiki/Habitability_of_red_dwarf_systems
M dwarf’s flare a lot, emit CME’s and the planets in the Habitable Zone are close in, tidally locked and hence have no magnetic field to protect their atmospheres.