New research suggests that the humble asteroid Vesta may have cracked open like an egg.
Continue reading “What Formed the Strange Troughs on Vesta?”Asteroid Bennu has little pieces of Vesta on it
The asteroid belt is a chaotic place. Things smash into each other, get thrown into completely different orbital planes, and are occasionally visited by small electronic spacecraft launched by humans. All three things seem to have happened to the asteroid Bennu, which is currently being orbited by OSIRIS-REx, a mission launched by NASA in 2016.
The most recently released results from the mission show that Bennu might have small pieces of Vesta on it. Given that Vesta is one of the biggest asteroid belt objects and Bennu is a near Earth asteroid millions of kilometers away from the asteroid belt, this hints at a pretty exciting past history for the asteroid currently being visited by NASA’s first asteroid sample return mission.
Continue reading “Asteroid Bennu has little pieces of Vesta on it”Space Dust Delivered Water to Vesta, Could it Have Done the Same for Earth?
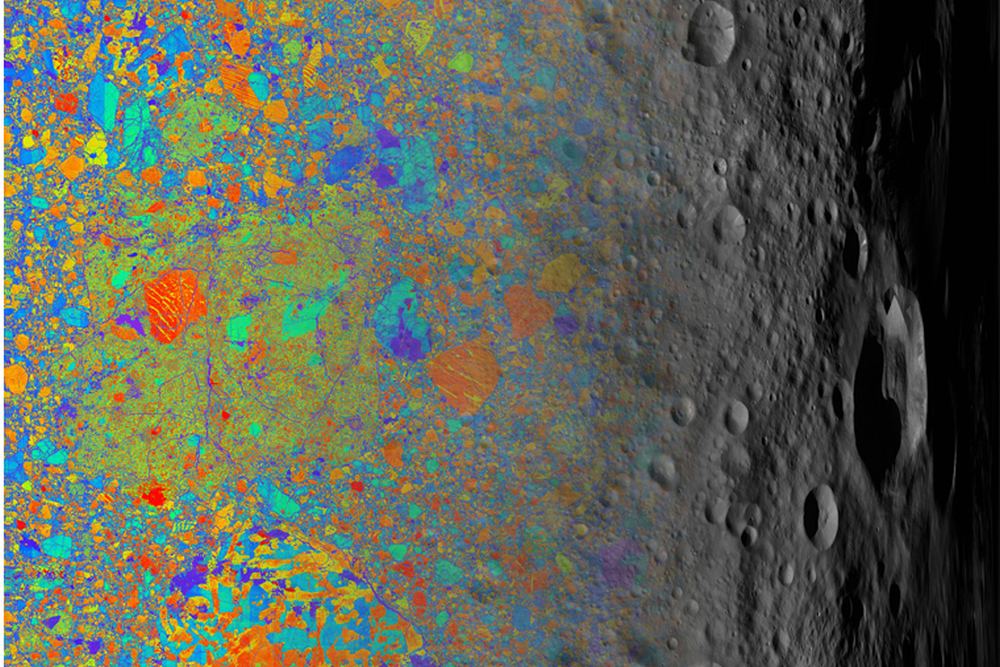
One of the most enduring questions about Earth regards the origins of its water. Where did it come from? One widely-held theory gives comets the honor of bringing water to Earth. Another one says that Earth’s water came when a protoplanet crashed into early Earth, not only delivering a vast quantity of water, but creating the Moon.
Now a new study shows that the minor planet Vesta got its water from space dust. Could that help explain the origin of Earth’s water?
Continue reading “Space Dust Delivered Water to Vesta, Could it Have Done the Same for Earth?”New Images of the “Golf Ball” Asteroid Pallas
In 1802, German astronomer Heinrich Olbers observed what he thought was a planet within the Main Asteroid Belt. In time, astronomers would come to name this body Pallas, an alternate name for the Greek warrior goddess Athena. The subsequent discovery of many more asteroids in the Main Belt would lead to Pallas being reclassified as a large asteroid, the third-largest in the Belt after Ceres and Vesta.
For centuries, astronomers have sought to get a better look at Pallas to learn more about its size, shape, and composition. As of the turn of the century, astronomers had come to conclude that it was an oblate spheroid (an elongated sphere). Thanks to a new study by an international team, the first detailed images of Pallas have finally been taken, which reveal that its shape is more akin to a “golf ball” – i.e. heavily dimpled.
Continue reading “New Images of the “Golf Ball” Asteroid Pallas”Ceres Rolled Over at Some Point in the Past

In 2007, the Dawn mission launched from Earth and began making its way towards two historic rendezvous in the Main Asteroid Belt. The purpose of this mission was to learn more about the history of the early Solar System by studying the two largest protoplanets in the Main Belt – Ceres and Vesta – which have remained intact since their formation.
In 2015, the Dawn mission arrived in orbit around Ceres and began sending back data that has shed light on the protoplanet’s surface, composition and interior structure. Based on mission data, Pasquale Tricarico – the senior scientist at the Planetary Science Institute (PSI) – has also determined that the Ceres also experienced an indirect polar reorientation in the past, where its pole rolled approximately 36° off-axis.
Continue reading “Ceres Rolled Over at Some Point in the Past”
Astro Challenge: Spotting 4 Vesta at its Best for Decades
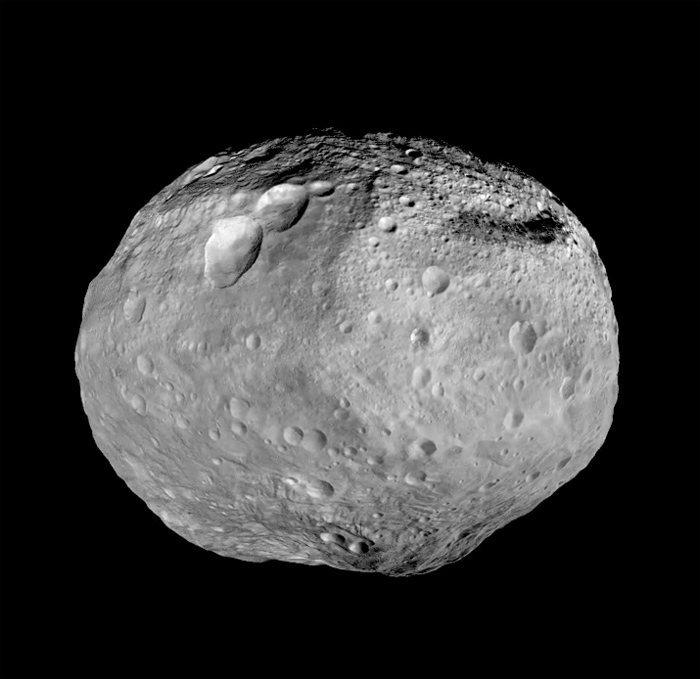
Up for a challenge? Planetary action is certainly heating up this summer: Jupiter passed opposition last month, Saturn does so in June, and Mars reaches favorable viewing next month. And with dazzling Venus in the west and Mercury to joining it starting in late June, we’ll soon have all of the naked eye classical planets in the evening sky.
Now, I want to turn your attention towards a potential naked eye object, one you’ve probably never seen: asteroid 4 Vesta.
Vistas of Vesta
Vesta reaches opposition in 2018 on the night of June 19th. At 1.14 Astronomical Units (AU, 170.8 million kilometers) distant, this year’s opposition is slightly more favorable than any other since 1989. We won’t get another pass nearly as close until May 2036. Vesta orbits the Sun once every 3.6 years, ranging from a perihelion of 2.15 AU to an aphelion of 2.57 AU.
Although Vesta was the fourth asteroid discovered, it’s actually the brightest, and the only one visible with the naked eye—that is, if you have dark skies, and know exactly where to look for it. This summer, Vesta loiters in the star rich realm of the astronomical constellation Sagittarius, “in the weeds” for viewers up north, but high in the sky for southern viewers.
Early June finds Vesta about 5 degrees northwest of the +3.8 magnitude star Mu Sagittarii, threading between the deep sky objects Messier 24 and Messier 25. Vesta then loops westward through the constellation Ophiuchus the Serpent Bearer starting on July 1st, before heading back to Sagittarius on September 5th.
Vesta in 2018
Catching Vesta with the naked eye isn’t easy. You’ll need dark rural skies with a limiting magnitude down to about +5.5, and a good beforehand knowledge of the fixed stars in the region. Vesta also spends 2018 weaving around the star-dappled plane of the Milky Way galaxy, making it an especially challenging target.
Binoculars or a telescope can bring the challenge within reach of suburban and urban skies, making it a pleasure to trace the track of Vesta from night to night. Sketch the background star field and you just might tease out the presence of Vesta as it slowly moves about 30′ arcminutes per night (the diameter of a Full Moon) through June. Crank up the magnification a bit using a large (10 inches aperture or greater) light bucket telescope, and you just might see the faint hint of an oblong disk… 348 by 277 miles (560 by 446 kilometers) in size, Vesta’s apparent size is 0.7” arcseconds around opposition, 1/3 the size of Neptune at its best.
The 99% illuminated, waxing gibbous Moon will actually occult 4 Vesta for Hawaii, Central America and the Galapagos Islands just eight days after opposition on the night of June 27th.
Discovered on the night of March 29th, 1807 by prolific asteroid hunter Heinrich Olber, the Hubble Space Telescope gave us our first blurry images of 4 Vesta back in 2007. NASA’s Dawn spacecraft gave us our first good views of Vesta as a world starting in mid- 2011, orbiting the potato-shaped asteroid for just over a year before departing for 1 Ceres in late 2012.
Attack of the Vestoid(s)
And did you know: we actually have identified samples of Vesta to study, right here on Earth. Vesta sustained a massive impact about a billion years ago, raining debris through the inner solar system. Dawn chronicled the resulting Rheasilvia impact basin on Vesta’s south pole, and asteroids such as 1981 Midas match the spectral composition of Vesta and are collectively known as “Vestoids”.
On Earth, meteorites such as QUE 97053 found in Antarctica and the 1913 Moore County fall in North Carolina also match up in composition to Vesta, and make up a subgroup known as Howardite-Eucrite-Diogenite (HED) meteorites. Collectively, space rocks from this single impact on 4 Vesta contribute to an amazing 5% of all the meteorites recovered on Earth.
Fascinating thoughts to ponder, as we follow the brightest asteroid through the summer sky.
New Study Sheds Light on How Earth and Mars Formed
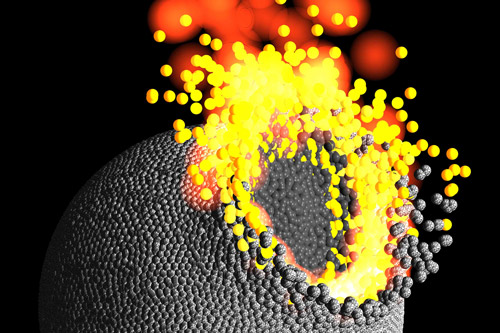
In accordance with the Nebular Hypothesis, the Solar System is believed to have formed through the process of accretion. Essentially, this began when a massive cloud of dust and gas (aka. the Solar Nebula) experienced a gravitational collapse at its center, giving birth to the Sun. The remaining dust and gas then formed into a protoplanetary disc around the Sun, which gradually coalesced to form the planets.
However, much about the process of how planets evolved to become distinct in their compositions has remained a mystery. Luckily, a new study by a team of researchers from the University of Bristol has approached the subject with a fresh perspective. By examining a combination of Earth samples and meteorites, they have shed new light on how planets like Earth and Mars formed and evolved.
The study, titled “Magnesium Isotope Evidence that Accretional Vapour Loss Shapes Planetary Compositions“, recently appeared in the scientific journal Nature. Led by Remco C. Hin, a senior research associate from the School of Earth Sciences at the University of Bristol, the team compared samples of rock from Earth, Mars, and the Asteroid Vesta to compare the levels of magnesium isotopes within them.
Their study attempted answering what has been a lingering question in the scientific community – i.e. did the planets form the way they are today, or did they acquire their distinctive compositions over time? As Dr. Remco Hin explained in a University of Bristol press release:
“We have provided evidence that such a sequence of events occurred in the formation of the Earth and Mars, using high precision measurements of their magnesium isotope compositions. Magnesium isotope ratios change as a result of silicate vapour loss, which preferentially contains the lighter isotopes. In this way, we estimated that more than 40 per cent of the Earth’s mass was lost during its construction. This cowboy building job, as one of my co-authors described it, was also responsible for creating the Earth’s unique composition.”
To break it down, accretion consists of clumps of material colliding with neighboring clumps to form larger objects. This process is very chaotic, and material is often lost as well as accumulated due to the extreme heat generated by these high-speed collisions. This heat is also believed to have created oceans of magma on the planets as they formed, not to mention temporary atmospheres of vaporized rock.
Until planets become about the same size as Mars, their force of gravitational attraction was too weak to hold onto these atmospheres. And as more collisions took place, the composition of these atmosphere and of the planets themselves would have changes substantially. How exactly the terrestrial planets – Mercury, Venus, Earth and Mars – obtained their current, volatile-poor compositions over time is what scientists have hoped to address.
For example, some believe that the planets current compositions are the result of particular combinations of gas and dust during the earliest periods of planet formation – where terrestrial planets are silicate/metal rich, but volatile poor, because of which elements were most abundant closest to the Sun. Others have suggested that their current composition is a consequence of their violent growth and collisions with other bodies.
To shed light on this, Dr. Hin and his associates analyzed samples of Earth, along with meteorites from Mars and the asteroid Vesta using a new analytical approach. This technique is capable of obtaining more accurate measurements of magnesium isotope rations than any previous method. This method also showed that all differentiated bodies – like Earth, Mars and Vesta – have isotopically heavier magnesium compositions than chondritic meteorites.
From this, they were able to draw three conclusions. For one, they found that Earth, Mars and Vesta have distinct magnesium isotope rations that could not be explained by condensation from the Solar Nebula. Second, they noted that the study of heavy magnesium isotopes revealed that in all cases, the planets lost about 40% percent of their mass during their formation period, following repeated episodes of vaporization.
Last, they determined that the accretion process results in other chemical changes that generate the unique chemical characteristics of Earth. In short, their study showed that Earth, Mars and Vesta all experiences significant losses of material after formation, which means that their peculiar compositions were likely the result of collisions over time. As Dr Hin added:
“Our work changes our views on how planets attain their physical and chemical characteristics. While it was previously known that building planets is a violent process and that the compositions of planets such as Earth are distinct, it was not clear that these features were linked. We now show that vapour loss during the high energy collisions of planetary accretion has a profound effect on a planet’s composition.”
Their study also indicated that this violent formation process could be characteristic of planets in general. These findings are not only significant when it comes to the formation of the Solar System, but of extra-solar planets as well. When it comes time to explore distant star systems, the distinctive compositions of their planets will tell us much about the conditions from which they formed, and how they came to be.
Further Reading: University of Bristol, Nature
Dawn Probe Finds Evidence of Subsurface Ice on Vesta
In 2011, NASA’s Dawn spacecraft established orbit around the large asteroid (aka. planetoid) known as Vesta. Over the course of the next 14 months, the probe conducted detailed studies of Vesta’s surface with its suite of scientific instruments. These findings revealed much about the planetoid’s history, its surface features, and its structure – which is believed to be differentiated, like the rocky planets.
In addition, the probe collected vital information on Vesta’s ice content. After spending the past three years sifting through the probe’s data, a team of scientists has produced a new study that indicates the possibility of subsurface ice. These findings could have implications when it comes to our understanding of how Solar bodies formed and how water was historically transported throughout the Solar System.
Their study, titled “Orbital Bistatic Radar Observations of Asteroid Vesta by the Dawn Mission“, was recently published in the scientific journal Nature Communications. Led by Elizabeth Palmer, a graduate student from Western Michigan University, the team relied on data obtained by the communications antenna aboard the Dawn spacecraft to conduct the first orbital bistatic radar (BSR) observation of Vesta.
This antenna – the High-Gain telecommunications Antenna (HGA) – transmitted X-band radio waves during its orbit of Vesta to the Deep Space Network (DSN) antenna on Earth. During the majority of the mission, Dawn’s orbit was designed to ensure that the HGA was in the line of sight with ground stations on Earth. However, during occultations – when the probe passed behind Vesta for 5 to 33 minutes at a time – the probe was out of this line of sight.
Nevertheless, the antenna was continuously transmitting telemetry data, which caused the HGA-transmitted radar waves to be reflected off of Vesta’s surface. This technique, known as bistatic radar (BSR) observations has been used in the past to study the surfaces of terrestrial bodies like Mercury, Venus, the Moon, Mars, Saturn’s moon Titan, and the comet 67P/CG.
But as Palmer explained, using this technique to study a body like Vesta was a first for astronomers:
“This is the first time that a bistatic radar experiment was conducted in orbit around a small body, so this brought several unique challenges compared to the same experiment being done at large bodies like the Moon or Mars. For example, because the gravity field around Vesta is much weaker than Mars, the Dawn spacecraft does not have to orbit at a very high speed to maintain its distance from the surface. The orbital speed of the spacecraft becomes important, though, because the faster the orbit, the more the frequency of the ‘surface echo’ gets changed (Doppler shifted) compared to the frequency of the ‘direct signal’ (which is the unimpeded radio signal that travels directly from Dawn’s HGA to Earth’s Deep Space Network antennas without grazing Vesta’s surface). Researchers can tell the difference between a ‘surface echo’ and the ‘direct signal’ by their difference in frequency—so with Dawn’s slower orbital speed around Vesta, this frequency difference was very small, and required more time for us to process the BSR data and isolate the ‘surface echoes’ to measure their strength.”
By studying the reflected BSR waves, Palmer and her team were able to gain valuable information from Vesta’s surface. From this, they observed significant differences in surface radar reflectivity. But unlike the Moon, these variations in surface roughness could not be explained by cratering alone and was likely due to the existence of ground-ice. As Palmer explained:
“We found that this was the result of differences in the roughness of the surface at the scale of a few inches. Stronger surface echoes indicate smoother surfaces, while weaker surface echoes have bounced off of rougher surfaces. When we compared our surface roughness map of Vesta with a map of subsurface hydrogen concentrations—which was measured by Dawn scientists using the Gamma Ray and Neutron Detector (GRaND) on the spacecraft—we found that extensive smoother areas overlapped areas that also had heightened hydrogen concentrations!”
In the end, Palmer and her colleagues concluded that the presence of buried ice (past and/or present) on Vesta was responsible for parts of the surface being smoother than others. Basically, whenever an impact happened on the surface, it transferred a great deal of energy to the subsurface. If buried ice was present there, it would be melted by the impact event, flow to the surface along impact-generated fractures, and then freeze in place.
Much in the same way that moon’s like Europa, Ganymede and Titania experience surface renewal because of the way cryovolcanism causes liquid water to reach the surface (where it refreezes), the presence of subsurface ice would cause parts of Vesta’ surface to be smoothed out over time. This would ultimately lead to the kinds of uneven terrain that Palmer and her colleagues witnessed.
This theory is supported by the large concentrations of hydrogen that were detected over smoother terrains that measure hundreds of square kilometers. It is also consistent with geomorphological evidence obtained from the Dawn Framing Camera images, which showed signs of of transient water flow over Vesta’s surface. This study also contradicted some previously-held assumptions about Vesta.
As Palmer noted, this could also have implications as far as our understanding of the history and evolution of the Solar System is concerned:
“Asteroid Vesta was expected to have depleted any water content long ago through global melting, differentiation, and extensive regolith gardening by impacts from smaller bodies. However, our findings support the idea that buried ice may have existed on Vesta, which is an exciting prospect since Vesta is a protoplanet that represents an early stage in the formation of a planet. The more we learn about where water-ice exists throughout the Solar System, the better we will understand how water was delivered to Earth, and how much was intrinsic to Earth’s interior during the early stages of its formation.”
This work was sponsored by NASA’s Planetary Geology and Geophysics program, a JPL-based effort that focuses on fostering the research of terrestrial-like planets and major satellites in the Solar System. The work was also conducted with the assistance of the USC’s Viterbi School of Engineering as part of an ongoing effort to improve radar and microwave imaging to locate subsurface sources of water on planets and other bodies.
Further Reading: USC, Nature Communications
How Far is the Asteroid Belt from the Sun?
In the 18th century, observations made of all the known planets (Mercury, Venus, Earth, Mars, Jupiter and Saturn) led astronomers to discern a pattern in their orbits. Eventually, this led to the Titius–Bode law, which predicted the amount of space between the planets. In accordance with this law, there appeared to be a discernible gap between the orbits of Mars and Jupiter, and investigation into it led to a major discovery.
Eventually, astronomers realized that this region was pervaded by countless smaller bodies which they named “asteroids”. This in turn led to the term “Asteroid Belt”, which has since entered into common usage. Like all the planets in our Solar System, it orbits our Sun, and has played an important role in the evolution and history of our Solar System.
Structure and Composition:
The Asteroid Belt consists of several large bodies, along with millions of smaller size. The larger bodies, such as Ceres, Vesta, Pallas, and Hygiea, account for half of the belt’s total mass, with almost one-third accounted for by Ceres alone. Beyond that, over 200 asteroids that are larger than 100 km in diameter, and 0.7–1.7 million asteroids with a diameter of 1 km or more.
Despite the impressive number of objects contained within the belt, the Main Belt’s asteroids are also spread over a very large volume of space. As a result, the average distance between objects is roughly 965,600 km (600,000 miles), meaning that the Main Belt consists largely of empty space. In fact, due to the low density of materials within the Belt, the odds of a probe running into an asteroid are now estimated at less than one in a billion.
The main (or core) population of the asteroid belt is sometimes divided into three zones, which are based on what is known as “Kirkwood gaps”. Named after Daniel Kirkwood, who announced in 1866 the discovery of gaps in the distance of asteroids, these gaps are similar to what is seen with Saturn’s and other gas giants’ systems of rings.
Origin:
Originally, the Asteroid Belt was thought to be the remnants of a much larger planet that occupied the region between the orbits of Mars and Jupiter. This theory was originally suggested by Heinrich Olbders to William Herschel as a possible explanation for the existence of Ceres and Pallas. However, this hypothesis has since been shown to have several flaws.
For one, the amount of energy required to destroy a planet would have been staggering, and no scenario has been suggested that could account for such events. Second, there is the fact that the mass of the Asteroid Belt is only 4% that of the Moon (and 22% that of Pluto). The odds of a cataclysmic collision with such a tiny body are very unlikely. Lastly, the significant chemical differences between the asteroids do no point towards a common origin.
Today, the scientific consensus is that, rather than fragmenting from an original planet, the asteroids are remnants from the early Solar System that never formed a planet at all. During the first few million years of the Solar System’s history, gravitational accretion caused clumps of matter to form out of an accretion disc. These clumps gradually came together, eventually undergoing hydrostatic equilibrium (become spherical) and forming planets.
However, within the region of the Asteroid Belt, planestesimals were too strongly perturbed by Jupiter’s gravity to form a planet. As such, these objects would continue to orbit the Sun as they had before, with only one object (Ceres) having accumulated enough mass to undergo hydrostatic equilibrium. On occasion, they would collide to produce smaller fragments and dust.
The asteroids also melted to some degree during this time, allowing elements within them to be partially or completely differentiated by mass. However, this period would have been necessarily brief due to their relatively small size. It likely ended about 4.5 billion years ago, a few tens of millions of years after the Solar System’s formation.
Though they are dated to the early history of the Solar System, the asteroids (as they are today) are not samples of its primordial self. They have undergone considerable evolution since their formation, including internal heating, surface melting from impacts, space weathering from radiation, and bombardment by micrometeorites. Hence, the Asteroid Belt today is believed to contain only a small fraction of the mass of the primordial belt.
Computer simulations suggest that the original asteroid belt may have contained mass equivalent to the Earth. Primarily because of gravitational perturbations, most of the material was ejected from the belt a million years after its formation, leaving behind less than 0.1% of the original mass. Since then, the size distribution of the asteroid belt is believed to have remained relatively stable.
When the asteroid belt was first formed, the temperatures at a distance of 2.7 AU from the Sun formed a “snow line” below the freezing point of water. Essentially, planetesimals formed beyond this radius were able to accumulate ice, some of which may have provided a water source of Earth’s oceans (even more so than comets).
Distance from the Sun:
Located between Mars and Jupiter, the belt ranges in distance between 2.2 and 3.2 astronomical units (AU) from the Sun – 329 million to 478.7 million km (204.43 million to 297.45 million mi). It is also an estimated to be 1 AU thick (149.6 million km, or 93 million mi), meaning that it occupies the same amount of distance as what lies between the Earth to the Sun.
The distance of an asteroid from the Sun (its semi-major axis) depends upon its distribution into one of three different zones based on the Belt’s “Kirkwood Gaps”. Zone I lies between the 4:1 resonance and 3:1 resonance Kirkwood gaps, which are roughly 2.06 and 2.5 AUs (3 to 3.74 billion km; 1.86 to 2.3 billion mi) from the Sun, respectively.
Zone II continues from the end of Zone I out to the 5:2 resonance gap, which is 2.82 AU (4.22 billion km; 2.6 mi) from the Sun. Zone III, the outermost section of the Belt, extends from the outer edge of Zone II to the 2:1 resonance gap, located some 3.28 AU (4.9 billion km; 3 billion mi) from the Sun.
While many spacecraft have been to the Asteroid Belt, most were passing through on their way to the outer Solar System. Only in recent years, with the Dawn mission, that the Asteroid Belt has been a focal point of scientific research. In the coming decades, we may find ourselves sending spaceships there to mine asteroids, harvest minerals and ices for use here on Earth.
We’ve written many articles about the Asteroid Belt here at Universe Today. Here’s What is the Asteroid Belt?, How Long Does it Take to get to the Asteroid Belt?, How Far is the Asteroid Belt from Earth?, Why Isn’t the Asteroid Belt a Planet?, and Why the Asteroid Belt Doesn’t Threaten Spacecraft.
To learn more, check out NASA’s Lunar and Planetary Science Page on asteroids, and the Hubblesite’s News Releases about Asteroids.
Astronomy Cast also some interesting episodes about asteroids, like Episode 55: The Asteroid Belt and Episode 29: Asteroids Make Bad Neighbors.
Sources:
How Long Does it Take to get to the Asteroid Belt?
Between the orbits of Mars and Jupiter lies the Solar System’s Main Asteroid Belt. Consisting of millions of objects that range in size from hundreds of kilometers in diameter (like Ceres and Vesta) to one kilometer or more, the Asteroid Belt has long been a source of fascination for astronomers. Initially, they wondered why the many objects that make it up did not come together to form a planet. But more recently, human beings have been eyeing the Asteroid Belt for other purposes.
Whereas most of our efforts are focused on research – in the hopes of shedding additional light on the history of the Solar System – others are looking to tap for its considerable wealth. With enough resources to last us indefinitely, there are many who want to begin mining it as soon as possible. Because of this, knowing exactly how long it would take for spaceships to get there and back is becoming a priority.
Distance from Earth:
The distance between the Asteroid Belt and Earth varies considerably depending on where we measure to. Based on its average distance from the Sun, the distance between Earth and the edge of the Belt that is closest to it can be said to be between 1.2 to 2.2 AUs, or 179.5 and 329 million km (111.5 and 204.43 million mi).
However, at any given time, part of the Asteroid Belt will be on the opposite side of the Sun, relative to Earth. From this vantage point, the distance between Earth and the Asteroid Blt ranges from 3.2 and 4.2 AU – 478.7 to 628.3 million km (297.45 to 390.4 million mi). To put that in perspective, the distance between Earth and the Asteroid Belt ranges between being slightly more than the distance between the Earth and the Sun (1 AU), to being the same as the distance between Earth and Jupiter (4.2 AU) when they are at their closest.
But of course, for reasons of fuel economy and time, asteroid miners and exploration missions are not about to take the long way! As such, we can safely assume that the distance between Earth and the Asteroid Belt when they are at their closest is the only measurement worth considering.
Past Missions:
The Asteroid Belt is so thinly populated that several unmanned spacecraft have been able to move through it on their way to the outer Solar System. In more recent years, missions to study larger Asteroid Belt objects have also used this to their advantage, navigating between the smaller objects to rendezvous with bodies like Ceres and Vesta. In fact, due to the low density of materials within the Belt, the odds of a probe running into an asteroid are now estimated at less than one in a billion.
The first spacecraft to make a journey through the asteroid belt was the Pioneer 10 spacecraft, which entered the region on July 16th, 1972 (a journey of 135 days). As part of its mission to Jupiter, the craft successfully navigated through the Belt and conducted a flyby of Jupiter (in December of 1973) before becoming the first spacecraft to achieve escape velocity from the Solar System.
At the time, there were concerns that the debris would pose a hazard to the Pioneer 10 space probe. But since that mission, 11 additional spacecraft have passed through the Asteroid Belt without incident. These included Pioneer 11, Voyager 1 and 2, Ulysses, Galileo, NEAR, Cassini, Stardust, New Horizons, the ESA’s Rosetta, and most recently, the Dawn spacecraft.
For the most part, these missions were part of missions to the outer Solar System, where opportunities to photograph and study asteroids were brief. Only the Dawn, NEAR and JAXA’s Hayabusa missions have studied asteroids for a protracted period in orbit and at the surface. Dawn explored Vesta from July 2011 to September 2012, and is currently orbiting Ceres (and sending back gravity data on the dwarf planet’s gravity) and is expected to remain there until 2017.
Fastest Mission to Date:
The fastest mission humanity has ever mounted was the New Horizons mission, which was launched from Earth on Jan. 19th, 2006. The mission began with a speedy launch aboard an Atlas V rocket, which accelerated it to a a speed of about 16.26 km per second (58,536 km/h; 36,373 mph). At this speed, the probe reached the Asteroid Belt by the following summer, and made a close approach to the tiny asteroid 132524 APL by June 13th, 2006 (145 days after launching).
However, even this pales in comparison to Voyager 1, which was launched on Sept. 5th, 1977 and reached the Asteroid Belt on Dec. 10th, 1977 – a total of 96 days. And then there was the Voyager 2 probe, which launched 15 days after Voyager 1 (on Sept. 20th), but still managed to arrive on the same date – which works out to a total travel time of 81 days.
Not bad as travel times go. At these speed, a spacecraft could make the trip to the Asteroid Belt, spend several weeks conducting research (or extracting ore), and then make it home in just over six months time. However, one has to take into account that in all these cases, the mission teams did not decelerate the probes to make a rendezvous with any asteroids.
Ergo, a mission to the Asteroid Belt would take longer as the craft would have to slow down to achieve orbital velocity. And they would also need some powerful engines of their own in order to make the trip home. This would drastically alter the size and weight of the spacecraft, which would inevitably mean it would be bigger, slower and a heck of a lot more expensive than anything we’ve sent so far.
Another possibility would be to use ionic propulsion (which is much more fuel efficient) and pick up a gravity assist by conducting a flyby of Mars – which is precisely what the Dawn mission did. However, even with a boost from Mars’ gravity, the Dawn mission still took over three years to reach the asteroid Vesta – launching on Sept. 27th, 2007, and arriving on July 16th, 2011, (a total of 3 years, 9 months, and 19 days). Not exactly good turnaround!
Proposed Future Methods:
A number of possibilities exist that could drastically reduce both travel time and fuel consumption to the Asteroid Belt, many of which are currently being considered for a number of different mission proposals. One possibility is to use spacecraft equipped with nuclear engines, a concept which NASA has been exploring for decades.
In a Nuclear Thermal Propulsion (NTP) rocket, uranium or deuterium reactions are used to heat liquid hydrogen inside a reactor, turning it into ionized hydrogen gas (plasma), which is then channeled through a rocket nozzle to generate thrust. A Nuclear Electric Propulsion (NEP) rocket involves the same basic reactor converting its heat and energy into electrical energy, which would then power an electrical engine.
In both cases, the rocket would rely on nuclear fission or fusion to generates propulsion rather than chemical propellants, which has been the mainstay of NASA and all other space agencies to date. According to NASA estimates, the most sophisticated NTP concept would have a maximum specific impulse of 5000 seconds (50 kN·s/kg).
Using this engine, NASA scientists estimate that it would take a spaceship only 90 days to get to Mars when the planet was at “opposition” – i.e. as close as 55,000,000 km from Earth. Adjusted for a distance of 1.2 AUs, that means that a ship equipped with a NTP/NEC propulsion system could make the trip in about 293 days (about nine months and three weeks). A little slow, but not bad considering the technology exists.
Another proposed method of interstellar travel comes in the form of the Radio Frequency (RF) Resonant Cavity Thruster, also known as the EM Drive. Originally proposed in 2001 by Roger K. Shawyer, a UK scientist who started Satellite Propulsion Research Ltd (SPR) to bring it to fruition, this drive is built around the idea that electromagnetic microwave cavities can allow for the direct conversion of electrical energy to thrust.
According to calculations based on the NASA prototype (which yielded a power estimate of 0.4 N/kilowatt), a spacecraft equipped with the EM drive could make the trip to Mars in just ten days. Adjusted for a trip to the Asteroid Belt, so a spacecraft equipped with an EM drive would take an estimated 32.5 days to reach the Asteroid Belt.
Impressive, yes? But of course, that is based on a concept that has yet to be proven. So let’s turn to yet another radical proposal, which is to use ships equipped with an antimatter engine. Created in particle accelerators, antimatter is the most dense fuel you could possibly use. When atoms of matter meet atoms of antimatter, they annihilate each other, releasing an incredible amount of energy in the process.
According to the NASA Institute for Advanced Concepts (NIAC), which is researching the technology, it would take just 10 milligrams of antimatter to propel a human mission to Mars in 45 days. Based on this estimate, a craft equipped with an antimatter engine and roughly twice as much fuel could make the trip to the Asteroid Belt in roughly 147 days. But of course, the sheer cost of creating antimatter – combined with the fact that an engine based on these principles is still theoretical at this point – makes it a distant prospect.
Basically, getting to the Asteroid Belt takes quite a bit of time, at least when it comes to the concepts we currently have available. Using theoretical propulsion concepts, we are able to cut down on the travel time, but it will take some time (and lots of money) before those concepts are a reality. However, compared to many other proposed missions – such as to Europa and Enceladus – the travel time is shorter, and the dividends quite clear.
As already stated, there are enough resources – in the form of minerals and volatiles – in the Asteroid Belt to last us indefinitely. And, should we someday find a way to cost-effective way to send spacecraft there rapidly, we could tap that wealth and begin to usher in an age of post-scarcity! But as with so many other proposals and mission concepts, it looks like we’ll have to wait for the time being.
We have written many articles about the asteroid belt for Universe Today. Here’s Where Do Asteroids Come From?, Why the Asteroid Belt Doesn’t Threaten Spacecraft, and Why isn’t the Asteroid Belt a Planet?.
Also, be sure to learn which is the Largest Asteroid in the Solar System, and about the asteroid named after Leonard Nimoy. And here’s 10 Interesting Facts about Asteroids.
We also have many interesting articles about the Dawn spacecraft’s mission to Vesta and Ceres, and asteroid mining.
To learn more, check out NASA’s Lunar and Planetary Science Page on asteroids, and the Hubblesite’s News Releases about Asteroids.
Astronomy Cast also some interesting episodes about asteroids, like Episode 55: The Asteroid Belt and Episode 29: Asteroids Make Bad Neighbors.
Sources: