Using nuclear devices to deflect or disrupt an asteroid. Sounds a bit crazy, no? Maybe a little too Hollywood? And yet, detonating nukes in space may be necessary someday for the sake of planetary defense. In order for this method to be effective, scientists need to work out all the particulars in advance. That means knowing how much force will be necessary depending on the mass and trajectory of the asteroid.
Recently, a research collaboration between Lawrence Livermore National Laboratory (LLNL) and the Air Force Institute of Technology (AFIT) investigated how the energy output of a nuclear detonation could affect the path of an asteroid. This consisted of modeling different nuclear reactions (fission or fusion) to determine the neutron energy generated, which could potentially pave the way for a new type of asteroid redirect mission (ARM).
Their research is described in a study, titled “Impact of neutron energy on asteroid deflection performance,” which was recently featured in the journal Acta Astronautica. The team behind it was led by Lansing Horan IV and colleagues from the Air Force Institute of Technology (AFIT), who conducted the research as part of a collaboration with the Weapons and Complex Integration Principal Directorate at the LLNL.
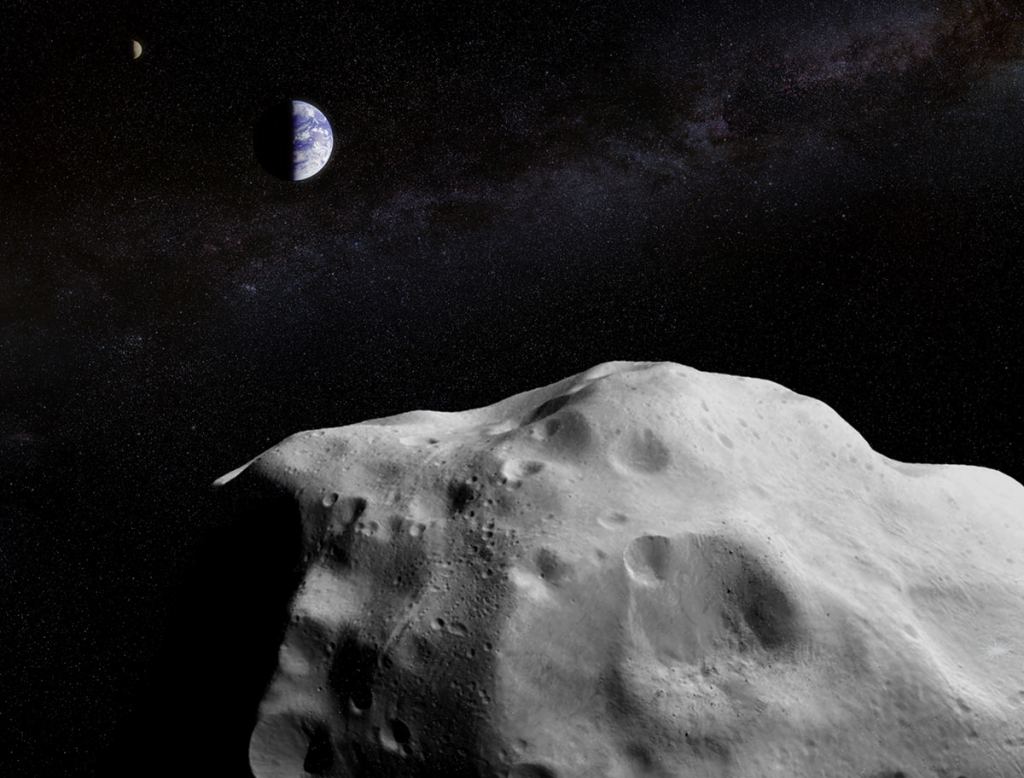
For the sake of their study, the team focused on neutron radiation produced by two different types of nuclear detonations – fission (an atomic bomb) and fusion (a thermonuclear bomb). The reason for this was that neutrons can be more penetrative than X-rays, another form of radiation produced by a nuclear detonation. In addition, neutrons of different energies can interact with the same matter through different mechanisms.
Deflection vs. Disruption
By comparing these two types of nuclear reactions side-by-side, the team was able to get a better idea of what types of neutron energies would be better for the sake of planetary defense. Essentially, there are two options when it comes to defeating an asteroid: disruption or deflection. As Horan explained in a recent LLNL news release, disruption consists of imparting so much energy into an asteroid that it is shattered into many fragments:
“This means that a neutron yield can potentially heat greater amounts of ‘asteroid surface material, and therefore be more effective for deflecting asteroids than an X-ray yield.”
“Past work found that more than 99.5 percent of the original asteroid’s mass would miss the Earth. This disruption path would likely be considered if the warning time before an asteroid impact is short and/or the asteroid is relatively small.”
Deflection, in contrast, is a gentler approach that involves imparting a smaller amount of energy to the asteroid in order to push it off course – leaving it otherwise intact. Similarly, nuclear devices are designed to produce different energy yields, where fission explosions are measured in terms of kilotons (kt), and fusion explosions are measured in terms of megatons (Mt).
With the right timing and calculations, even a small amount of energy could deflect an asteroid well in advance. As Horan summarized:
“Over time, with many years prior to impact, even a minuscule velocity change could add up to an Earth-missing distance. Deflection might generally be preferred as the safer and more ‘elegant’ option, if we have sufficient warning time to enact this sort of response. This is why our work focused on deflection.”
Running the Numbers
In order to determine which option was best, the team divided their research into two primary phases, which included neutron energy deposition and asteroid deflective response. The first phase was conducted using the Monte Carlo N-Particle (MCNP) radiation-transport code, which was developed by the Los Alamos National Laboratory to track how different particles behave over a broad range of energies.
Using MCNP, the team considered a number of energy-deposition scenarios involving a spherical asteroid 300 meters (985 ft) in diameter and composed of silicon oxide (SiO2). This asteroid was divided into hundreds of concentric spheres and cones to create hundreds of thousands of cells. They then considered how radiating neutrons would deposit energy onto this asteroid and how it would be distributed throughout its interior.
The second phase, which relied on the LLNL’s 3D Arbitrary Lagrangian-Eulerian (ALE3D) hydrodynamics code, consisted of simulating how the asteroid’s material would respond to the different energy depositions under consideration. The MCNP profiles were then imported and integrated with the ALE3D asteroid and the simulations were performed.
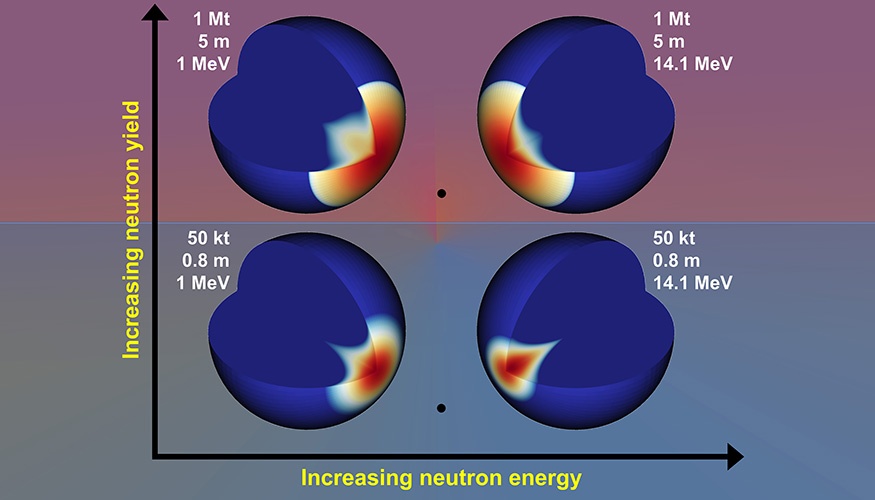
What they found was that different energy deposition profiles led to drastically different changes in an asteroid’s direction and velocity, indicating that it is the primary factor (rather than spatial distribution). They also concluded that deflection was preferable to disruption and that precision and accuracy were paramount, especially where large yields used to deflect large asteroids were concerned.
As Horan indicated, while there’s much more research to be done, their work is a step in the direction of nuclear deflection simulations. When it comes time to plan an asteroid mitigation mission, the ability to account for these energy parameters will be paramount to success:
“It is important that we further research and understand all asteroid mitigation technologies in order to maximize the tools in our toolkit. In certain scenarios, using a nuclear device to deflect an asteroid would come with several advantages over non-nuclear alternatives. In fact, if the warning time is short and/or the incident asteroid is large, a nuclear explosive might be our only practical option for deflection and/or disruption.”
This collaborative research was conducted as part of Horan’s nuclear engineering master’s program at AFIT. He was joined by Darren E. Holland and James E. Bevins, a Research Assistant Professor and Assistant Professor in Nuclear Engineering at AFIT (respectively). Their co-authors included Megan Bruck Syal and Joseph Wasem from LLNL’s Weapons and Complex Integration Principal Directorate.
Further Reading: LLNL, Acta Astronautica