During the Apollo Era, astronauts conducted vital science operations on the Moon, which included bringing samples of lunar rocks back to Earth for study. Thanks to the examination of these rocks, scientists were able to learn a great deal about the formation and evolution of the Moon and even found evidence of lunar water. In the coming years, when NASA sends astronauts back as part of Project Artemis, more samples will be returned.
Recently, NASA put out the call for science white papers to help them design a framework for the kind of science operations the Artemis astronauts will conduct. According to one proposal, the Artemis astronauts should not only bring back samples of lunar regolith or rocks but lunar ice as well. By examining them here on Earth, scientists may finally be able to resolve the mystery of where the Moon’s water came from.
This proposal was the subject of a white paper submitted to the Artemis Science Definition Call, titled “Lunar Near-Surface Volatile Sample Return.” The lead author on the paper was Igor Aleinov, an associate research scientist at Columbia University’s Center for Climate Systems Research (CCSR) and NASA’s Goddard Institute for Space Sciences (GISS).
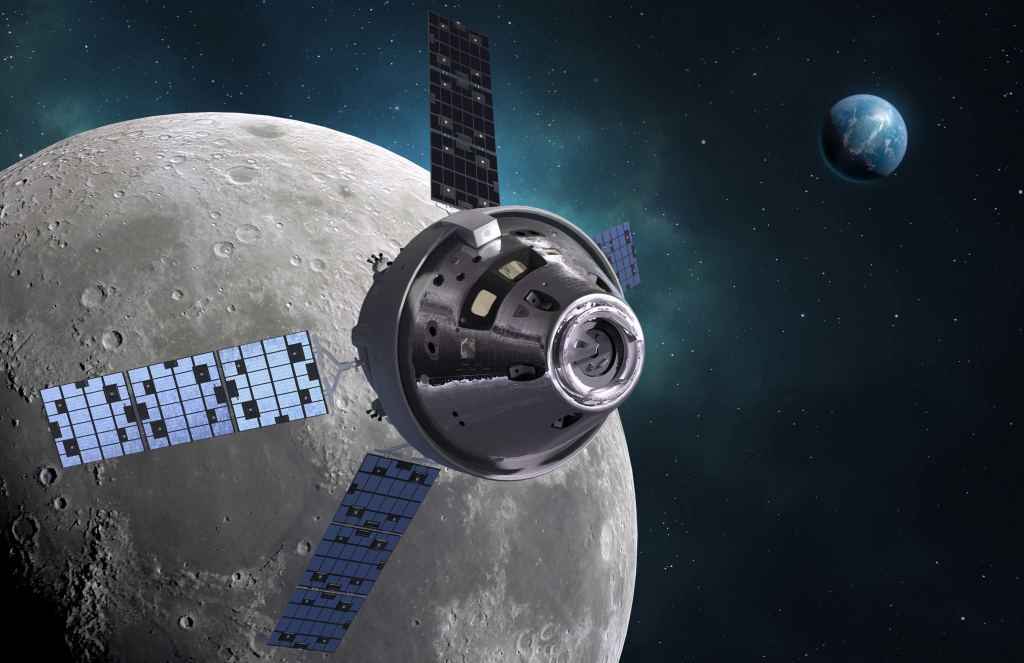
To break it down, scientists have speculated that ice could exist on the Moon since the days of the Space Race. This ice, they argued, could have been deposited by comets or could be produced by interactions between hydrogen (from solar wind) and oxygen-rich rocks. It was not until the 1990s, and the Lunar Prospector spacecraft, that the first clear evidence of lunar water was discovered.
Launching in 1998, this NASA mission spent over a year and a half mapping the entire surface of the Moon and detected high concentrations of hydrogen in the regolith near the polar regions within a depth of one meter (~3.3 ft). These findings were confirmed by India’s Chandrayaan-1 orbiter in 2008 and the Lunar Reconnaissance Orbiter (LRO) in 2009, particularly in the permanently shadowed craters in the Moon’s South Pole-Aitken Basin.
Shortly thereafter, the Lunar Crater Observation and Sensing Satellite (LCROSS) was sent to the Moon and impacted in the southern Cabeus crater. Spectra obtained from the resulting debris showed concentrations of water that were up to ~5% by weight, plus an abundance of other volatiles like hydrogen sulfide (H2S), carbon monoxide (CO), and ammonia (NH3).
However, the origin, distribution, depth, and volume of lunar water remain unanswered questions. For example, scientists are unsure whether solar wind, volatile-rich impactors, or volcanic outgassing is chiefly responsible. Addressing them has become even more important with all the proposals to build human outposts in the South Pole-Aitken Basin in the near future.
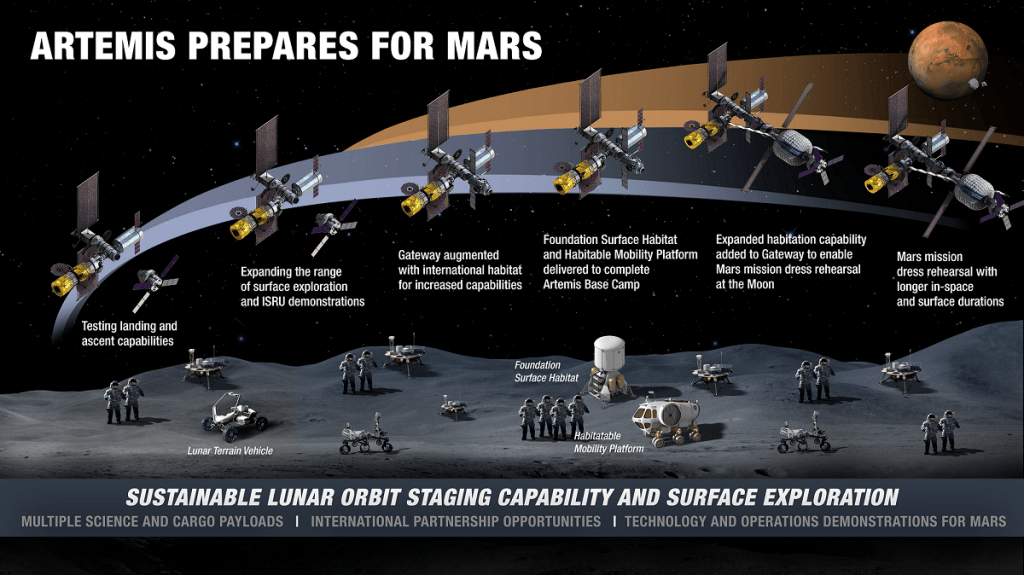
As Aleinov told Universe Today via email:
“To have a reliable (and economically feasible) source of water is crucial for a long-term human outpost on the Moon. One needs water for everyday human activity and for production of food. But what is even more important, one can use water as a source of rocket fuel by splitting it into hydrogen and oxygen. Knowing the origin of lunar water will help people to search for its sources more efficiently.
“For example, if it is of volcanic origin, we will need to look into older craters and dig to sufficient depth (2-5 meters). If it was delivered by comets, then the age of the crater is less important and we can probe crates at random looking for water close to the surface, which would be easier to mine.
“Finally, if the main source of water is solar wind, then it is more likely to be distributed uniformly over the shadowed craters. But its concentration in lunar regolith will be lower than in the other two cases. So, in this case, it would make more sense to invest more resources into efficient mining technologies rather than searching for rich deposits.”
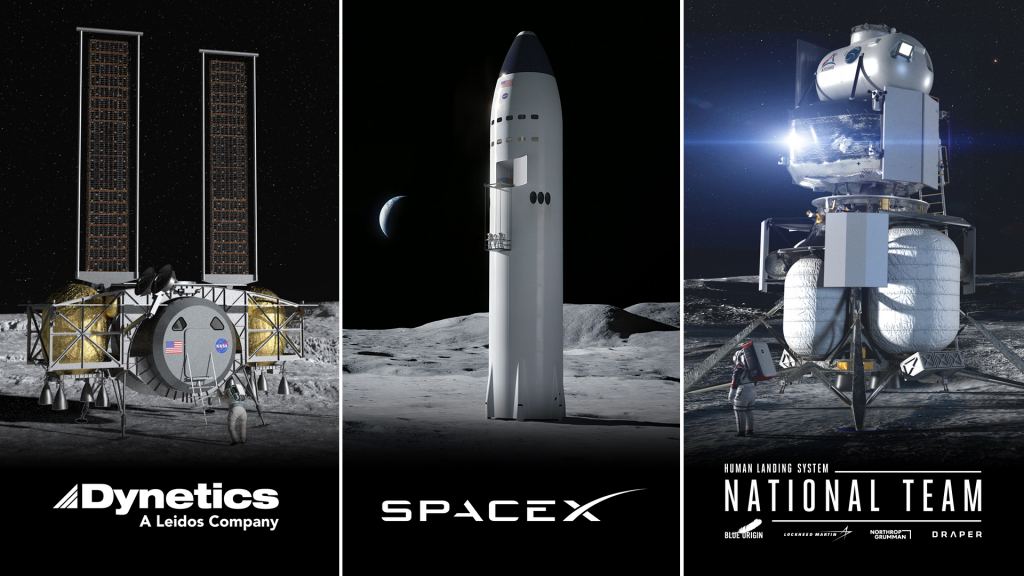
Addressing these mysteries are things Aleinov and his colleagues hope an Artemis mission, which could include a lunar ice sample-return, will address. For starters, they hope in addition to its source, we will be able to learn why it is distributed the way it is on the Moon. On Mercury, which also has PSRs in its polar regions, water ice is evenly distributed within its craters. As Aleinov explained, the Moon is a different case:
“Not all shadowed craters have surface water. Where it is observed it is patchy and less abundant. But we do observe substantial amounts of subsurface water through the neutron spectroscopy. So, the story of the Moon’s water is more complicated. Did it come from different sources than on Mercury? Why do some otherwise similar craters have surface water while others do not? How much water do we actually have on the Moon? We still don’t have satisfactory answers to these questions.”
The reason these questions have remained unanswered comes down to a simple problem of access. All of the lunar samples collected by the Apollo astronauts and robotic sample-return missions have come from locations far away from the poles. As a result, all of the data we have on the Moon’s water comes from remote sensing.
But beyond the location and spatial distribution of water patches, says Aleinov, there’s so much that we don’t know about the Moon’s water. This includes its vertical distribution (i.e. whether or not it exists below a 1-meter depth) or it’s isotopic content, which would differ depending on the source. In short, we don’t have the data we need to determine the source or the abundance of lunar water.

Here’s where Project Artemis can make an even bigger impact. In addition to their regular science operations on the lunar surface (which will include sample returns), the astronauts participating in the Artemis III mission (or later crewed missions) will be able to investigate and procure lunar ice samples to known depths.
This, says Aleinov, will allow for scientists on Earth to learn the timing of the water’s deposition and its isotopic content, which will let them known where it came from:
“Of particular interest will be samples from deeper (2-5 m) layers of regolith. The samples from such depth represent undisturbed deposits from ~3.5 billion years ago. This is a period of peak volcanic activity and such samples will tell us how much of volcanic water was actually delivered to the polar regions and if this is a primary source of lunar water.
“Also, the distribution of water in such samples and concentrations of other volatiles will tell us a lot about the evolution of the Moon at that period. In particular, we will understand better the power and frequency of volcanic eruptions, what kind of atmosphere they could form, and what could be the role of such a transient atmosphere in the Moon’s history.”

This and other proposals are currently being reviewed by NASA’s Science Definition Team (SDT), a body formed by the Planetary Science Division of NASA’s Science Mission Directorate (SMD). The call for white papers opened on October 25th, 2019, and closed earlier this month (on Sept. 8th). When astronauts return to the Moon in 2024 (Artemis III) some of these proposals will be included in their list of activities.
The co-authors on the study included Michael J. Way, a researcher with the Goddard Institute for Space Sciences; Christopher W. Hamilton of the Lunar and Planetary Lab at the University of Arizona; and James W. Head, the Louis and Elizabeth Scherck Distinguished Professor of Geological Sciences at Brown University.
Further Reading: arXiv