NASA’s Spitzer Space Telescope may be retired, but the things it witnessed during its sixteen and a half year mission will be the subject of study for many years to come. For instance, Spitzer is the only telescope to witness something truly astounding occurring at the center of the distant galaxy OJ 287: a supermassive black hole (SMBH) orbited by another black hole that regularly passes through its accretion disk.
Whenever this happens, it causes a flash that is brighter than all the stars in the Milky Way combined. Using Spitzer‘s observations, an international team of astronomers was able to finally create a model that accurately predicts the timing of these flashes and the orbit of the smaller black hole. In addition to demonstrating General Relativity in action, their findings also provide validation to Stephen Hawking‘s “no-hair theorem.”
Located 3.5 billion light-years from Earth, OJ 287 is what is known as a “blazar,” a galaxy with a particularly active nucleus and a jet of high-energy particles extending from its center. At its center is an SMBH that is roughly 18 billion times as massive as our Sun, making it the largest ever discovered – whereas the black hole at the center of the Milky Way (Sagittarius A*) is about 4 million Solar masses.
For decades, astronomers have been aware of the binary nature of this black hole and determined that its companion – which is 150 million times as massive as our Sun – completes an orbit once every 12 years. Twice in its orbit, the smaller black hole crashes through the massive disk of gas and dust surrounding its larger companion. These collisions create expanding bubbles of hot gas that move away from the disk and are extremely bright.
These flares are known as Eddington flares, which were so-named to commemorate the centennial of the solar eclipse observations conducted by Sir Arthur Eddington in 1919. This famous campaign validated predictions made by Albert Einstein and his Theory of General Relativity, which he had formalized just four years prior.
Because of the irregular orbit of the smaller black hole, it shifts position with each 12-year orbit and is tilted relative to the accretion disk. This means that it collides with the disk and create flares at different times during its cycle. As long as astronomers have known about the binary nature of these black holes, there have been attempts to create a model that could accurately predict the occurrence of these flares.
In 2010, scientists succeeded for the first time with a model that could predict these flares to within one to three weeks, which was confirmed when they predicted the appearance of a flare in December 2015 to within three weeks. By 2018, the same team responsible for this latest study released an even more accurate model that could predict a flash to within four hours.
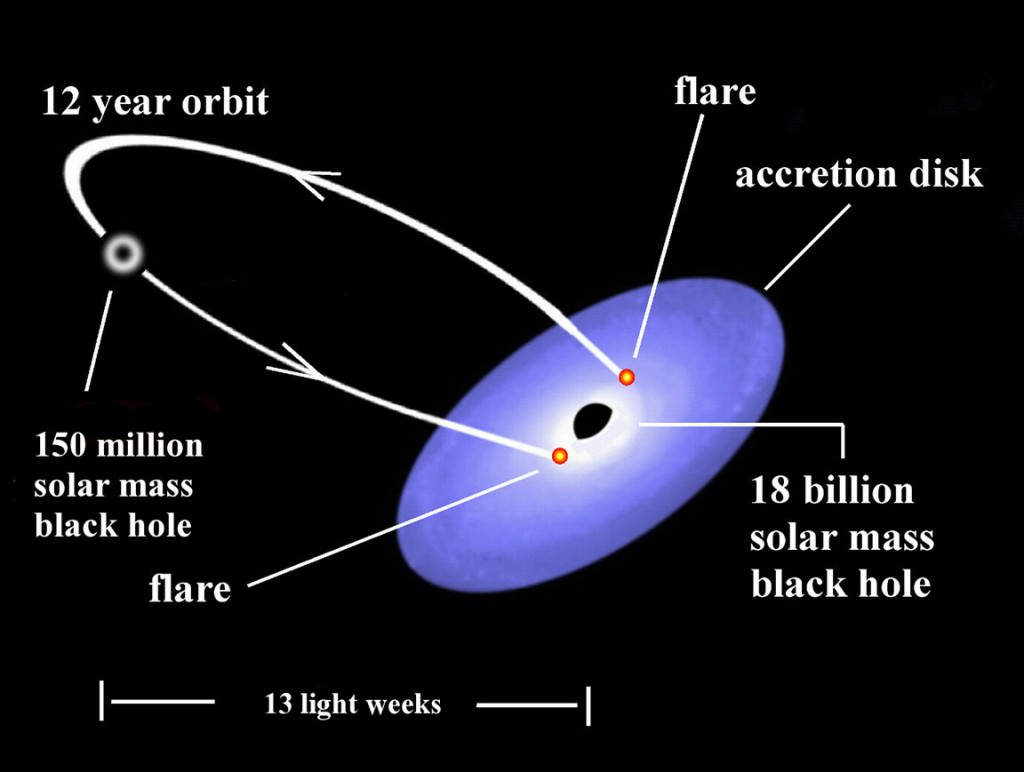
In their latest study, the team showed how Spitzer data confirms that their model is correct because of how it accurately predicted a flare that occurred on July 31st, 2019. The paper that describes their findings, titled “Spitzer Observations of the Predicted Eddington Flare from Blazar OJ 287“, recently appeared in the Astrophysical Journal Letters.
The observations were rather fortuitous, as OJ 287 was on the opposite side of the Sun at the time. Luckily, Spitzer’s wide orbit – which brings it to a maximum distance of 254 million km (158 million mi) from Earth – allowed it to observe the galaxy on July 31st, the same day a flare was expected. Spitzer was able to keep observing the galaxy until September, at which point, the galaxy was no longer obscured by the Sun.
Seppo Laine, an associate staff scientist at Caltech/IPAC and the lead author on the team’s study, was responsible for overseeing Spitzer’s observations of the system. As he stated in a recent NASA press release:
“When I first checked the visibility of OJ 287, I was shocked to find that it became visible to Spitzer right on the day when the next flare was predicted to occur. It was extremely fortunate that we would be able to capture the peak of this flare with Spitzer, because no other human-made instruments were capable of achieving this feat at that specific point in time.”
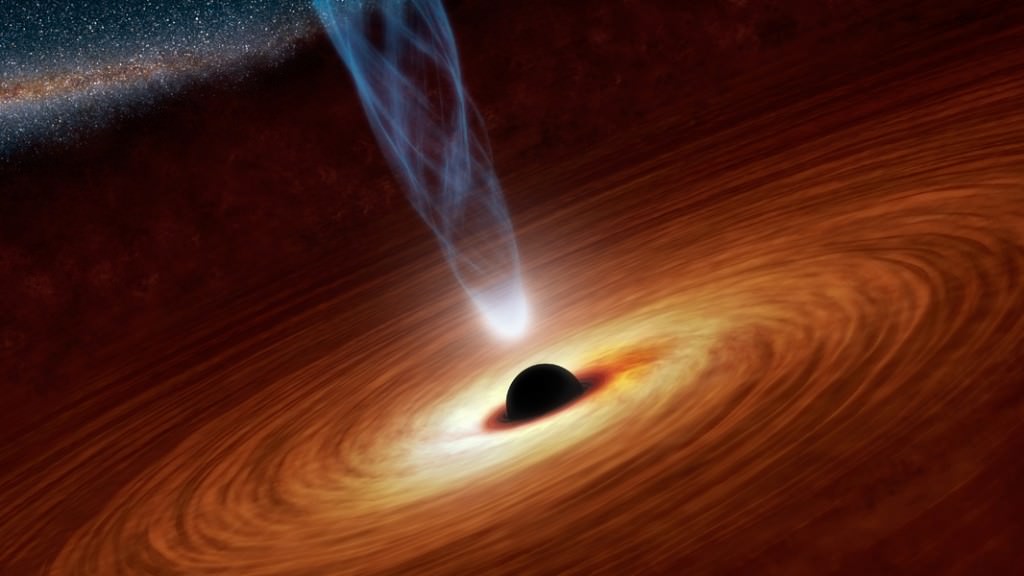
The nature of OJ 287 is also expected to produce gravity waves that astronomers here on Earth will be able to detect using facilities like the Laser Interferometer Gravitational Wave Observatory (LIGO). In fact, the waves created by this system are expected to be so large and energetic that they will measurably alter the smaller black hole’s orbit (and hence, the timing of the flares).
Previous studies have been made of OJ 287 that have accounted for gravitational waves, but the 2018 model is the most detailed to date. By factoring information gained by LIGO since 2015 into this model, the team was able to narrow the window in which a flare is expected to just 1.5 days. To further refine their predictions, they also included details about the larger black hole’s physical characteristics.
Specifically, the new model incorporates the “no-hair” theorem of black holes, a theory originally proposed in the 1960s by a team of physicists that included Stephen Hawking. This theorem predicts that the “surface” of a black hole – or rather, their outer boundary (aka. event horizon) – is entirely symmetrical along its rotational axis. This further narrowed the team’s predictive model to just a few hours.
By predicting the smaller black hole’s orbit with this level of precision, the new model supports the no-hair theorem. This confirms yet another prediction, this one made by Caltech professor emeritus Kip Thorne, who in the 1970s described how an object orbiting an SMBH could reveal whether its surface was symmetrical or “hairy.”
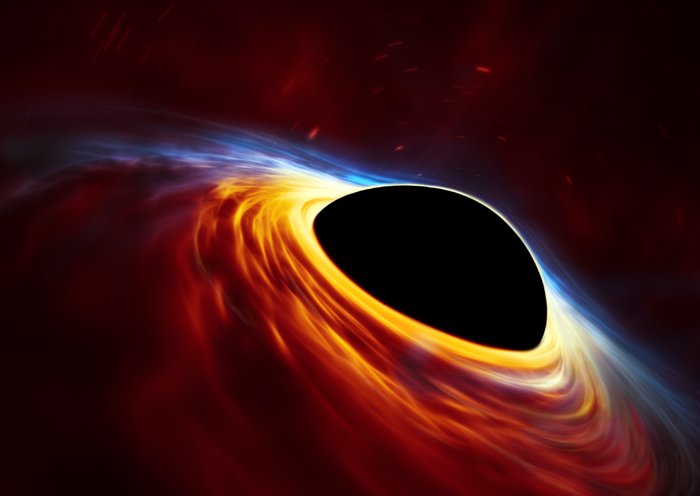
Accounting for whether or not a black hole is smooth and symmetrical is important to determine the timing of the smaller black hole’s orbit. While an object’s orbit is predominantly a question of mass, the distribution of that mass matters as well. So if a black hole were “hairy”, it would have a noticeable effect on the orbit of anything around it.
In short, the OJ 287 system supports the idea that black hole surfaces are symmetrical along their rotational axes. “It is important to black hole scientists that we prove or disprove the no-hair theorem,” said Mauri Valtonen, an astrophysicist from the University of Turku and a coauthor on the paper. “Without it, we cannot trust that black holes as envisaged by Hawking and others exist at all.”
Such is the nature of missions like Spitzer. Long after they retire, the results they obtained over their history of service continue to inspire discoveries and breakthroughs. So long Spitzer, and thanks for all the infrared data on the cosmos!
Further Reading: NASA
There’s a typo at the end of the third paragraph, “…whereas the black hole at the center of the Milky Way (Sagittarius A*) is about 40 Solar masses.”
Should be 4 million Solar masses.
This is a result of great importance, based on great serendipity and great work; it is truly great^3!
The serendipity is in 1) being able to use Spitzer’s last weeks when no ground based observatory could do it, as well as 2) having the smaller black hole orbit through the disk with two pass close together [see their fig. 1] allowing ground based optical calibration of near-IR Spitzer data after the flare peak [!: visibility and scheduling issues]; and 3) having the disk pass flare being a flat bremsstrahlung spectrum as no other flare (usually power-law spectra).
The work is in preparing Spitzer and the complicated GR analysis.
The importance is many-fold.
– This – the “no hair” membrane effects on the gravitational wave spectra and the flat bremssstrahlung – are the first observations of a unique black hole property and its unique disk pass property respectively, thus rejecting all other objects.
– It is also the first observation of general relativity up to the very event horizon of a black hole, confirming general relativity for all scales of cosmology. The earlier, implicit evidence has been from the EHT image of M87* black hole, where the innermost stable circular orbit was successfully predicted from linearized GR and will – in extremal black holes spinning at universal speed limit – overlap with the event horizon.
– Apart from the complicated retardation effects of the gravitational near field coupling to the far field gravitational waves (and the orbit precession, say) which contribute small nonlinearities, apparently all the general relativity description we need up to the event horizon is linearized. E.g. based on Minkowski flat space and small deviations thereof [see their reference to the 1980 tome of Kip Thorne on gravitational radiation: https://authors.l…p80a.pdf ].
“While an object’s orbit is predominantly a question of mass, the distribution of that mass matters as well.”
In general relativity, yes, because this effects the gravitational wave coupling. Any other surface than a “no hair” event horizon couple at least twice as much, so that makes for a faster precession I think.
On the other hand we should not discount the general relativistic precession effect, since that is what has kept the solar system stable over 4.5 billion years [ http://www.scholarpedia.org/article/Stability_of_the_solar_system ]!