The full, weird story of the quantum world is much too large for a single article, but the period from 1905, when Einstein first published his solution to the photoelectric puzzle, to the 1960’s, when a complete, well-tested, rigorous, and insanely complicated quantum theory of the subatomic world finally emerged, is quite the story.
Continue reading “Why Quantum Mechanics Defies Physics”Thinking About Time Travel Helps Solve Problems in Physics
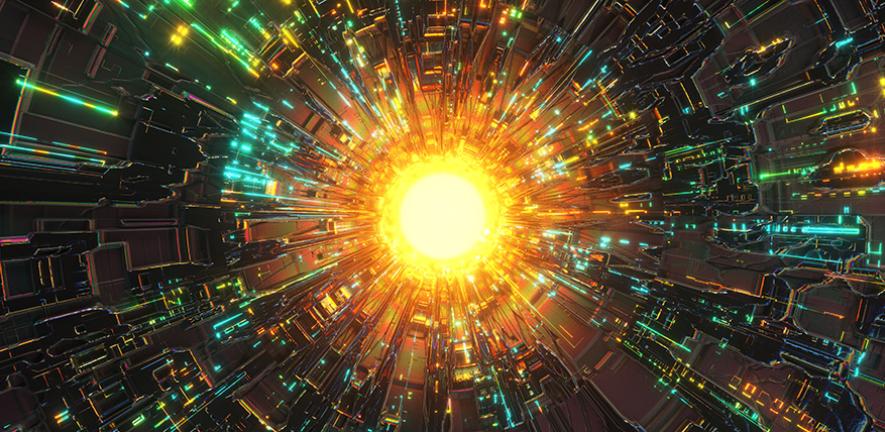
Time travel. We’ve all thought about it at one time or another, and the subject has been explored extensively in science fiction. Once in a while, it is even the subject of scientific research, typically involving quantum mechanics and how the Universe’s four fundamental forces (electromagnetism, weak and strong nuclear forces, and gravity) fit together. In a recent experiment, researchers at the University of Cambridge showed that by manipulating quantum entanglements, they could simulate what could happen if the flow of time were reversed.
Continue reading “Thinking About Time Travel Helps Solve Problems in Physics”New Muon g-2 Result Improves the Measurement by a Factor of 2
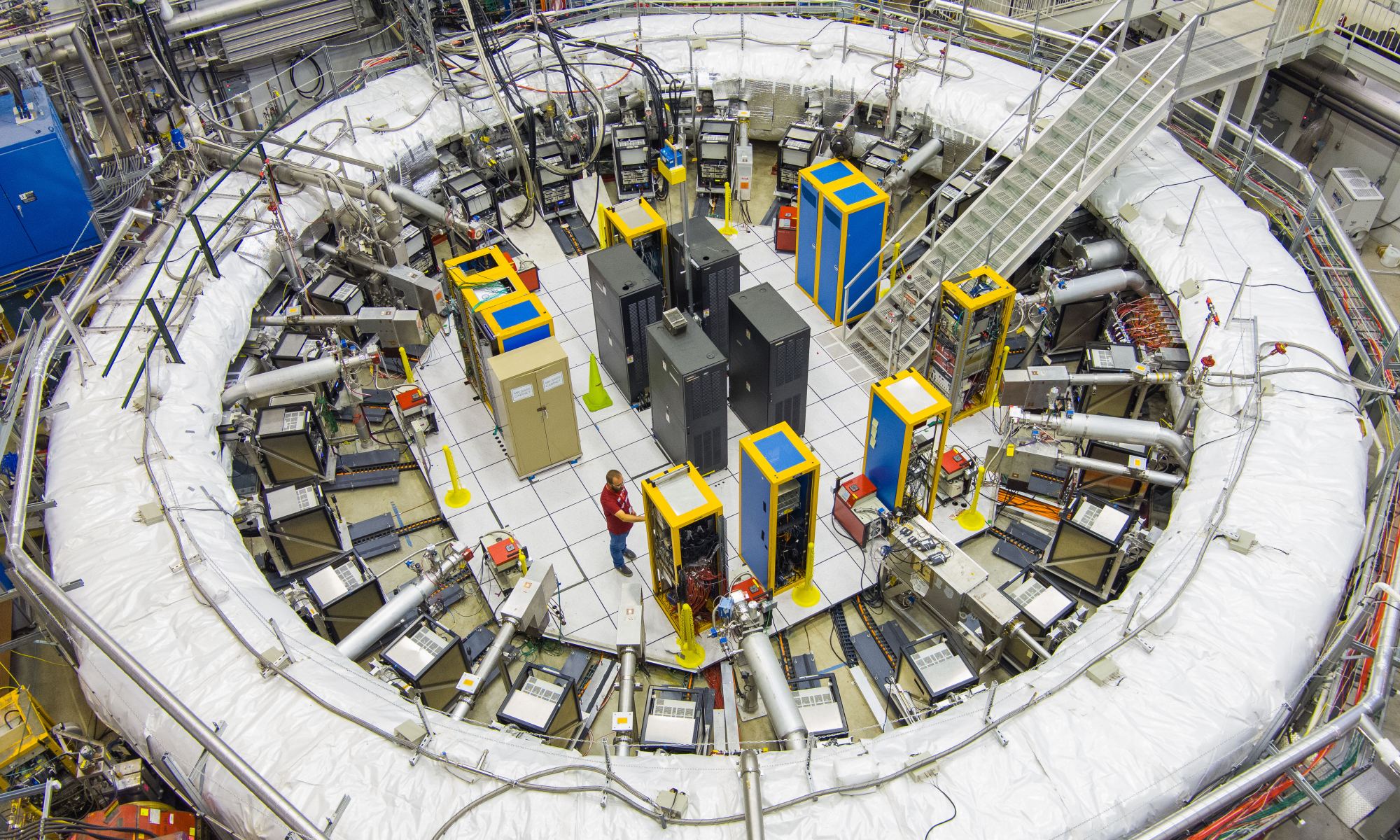
At the Fermi National Accelerator Laboratory (aka. Fermilab), an international team of scientists is conducting some of the most sensitive tests of the Standard Model of Particle Physics. The experiment, known as Muon g-2, measures the anomalous magnetic dipole moment of muons, a fundamental particle that is negatively charged (like electrons) but over 200 times as massive. In a recent breakthrough, scientists at Fermilab made the world’s most precise measurement of the muon’s anomalous magnetic moment, improving the precision of their previous measurements by a factor of 2.
Continue reading “New Muon g-2 Result Improves the Measurement by a Factor of 2”Are We Entering the Era of Quantum Telescopes?
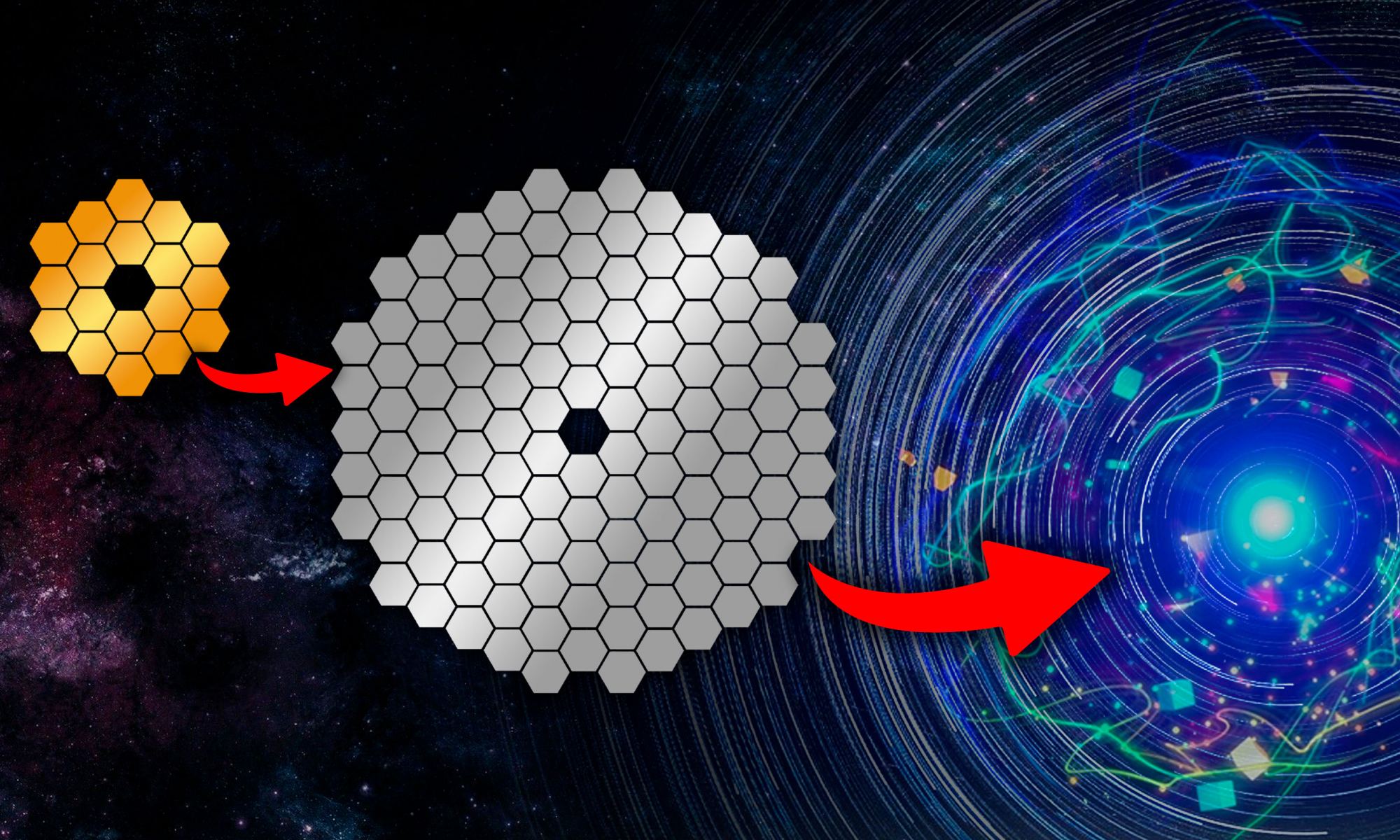
For astronomers, one of the greatest challenges is capturing images of objects and phenomena that are difficult to see using optical (or visible light) telescopes. This problem has been largely addressed by interferometry, a technique where multiple telescopes gather signals, which is then combined to create a more complete picture. Examples include the Event Horizon Telescope, which relies on observatories from around the world to capture the first images of the supermassive black hole (SMBH) at the center of the M87 galaxy, and of Sagittarius A* at the center of the Milky Way.
That being said, classic interferometry requires that optical links be maintained between observatories, which imposes limitations and can lead to drastically increased costs. In a recent study, a team of astrophysicists and theoretical physicists proposed how these limitations could be overcome by relying on quantum mechanics. Rather than relying on optical links, they propose how the principle of quantum entanglements could be used to share photons between observatories. This technique is part of a growing field of research that could lead to “quantum telescopes” someday.
Continue reading “Are We Entering the Era of Quantum Telescopes?”Do Advanced Civilizations use Black Holes as Giant Quantum Computers?
If life is common in our Universe, and we have every reason to suspect it is, why do we not see evidence of it everywhere? This is the essence of the Fermi Paradox, a question that has plagued astronomers and cosmologists almost since the birth of modern astronomy. It is also the reasoning behind the Hart-TIpler Conjecture, one of the many (many!) proposed resolutions, which asserts that if advanced life had emerged in our galaxy sometime in the past, we would see signs of their activity everywhere we looked. Possible indications include self-replicating probes, megastructures, and other Type III-like activity.
On the other hand, several proposed resolutions challenge the notion that advanced life would operate on such massive scales. Others suggest that advanced extraterrestrial civilizations would be engaged in activities and locales that would make them less noticeable. In a recent study, a German-Georgian team of researchers proposed that advanced extraterrestrial civilizations (ETCs) could use black holes as quantum computers. This makes sense from a computing standpoint and offers an explanation for the apparent lack of activity we see when we look at the cosmos.
Continue reading “Do Advanced Civilizations use Black Holes as Giant Quantum Computers?”Fermilab’s Muon g-2 Experiment Finally Gives Particle Physicists a Hint of What Lies Beyond the Standard Model
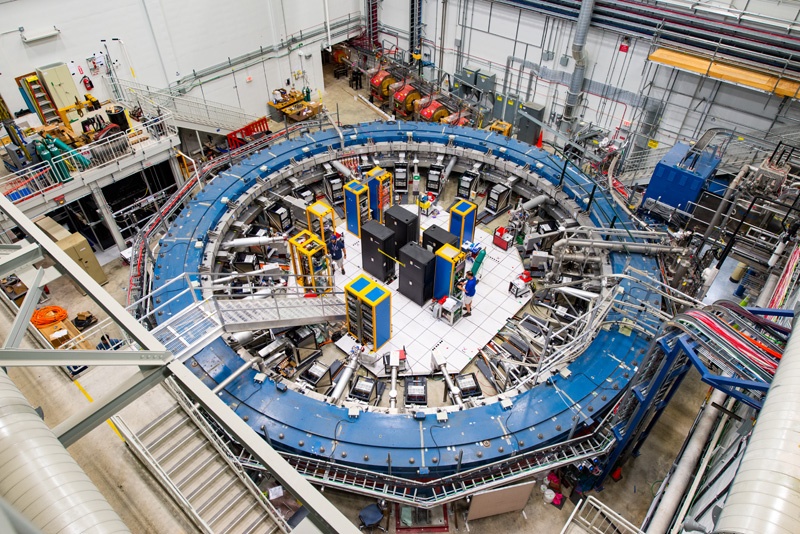
Since the long-awaited detection of the Higgs Boson in 2012, particle physicists have been probing deeper into the subatomic realm in the hope of investigating beyond the Standard Model of Particle Physics. In so doing, they hope to confirm the existence of previously unknown particles and the existence of exotic physics, as well as learning more about how the Universe began.
At the Fermi National Accelerator Laboratory (aka. Fermilab), researchers have been conducting the Muon g-2 experiment, which recently announced the results of their first run. Thanks to the unprecedented precision of their instruments, the Fermilab team found that muons in their experiment did not behave in a way that is consistent with the Standard Model, resolving a discrepancy that has existed for decades.
Continue reading “Fermilab’s Muon g-2 Experiment Finally Gives Particle Physicists a Hint of What Lies Beyond the Standard Model”Quantum Theory Proposes That Cause and Effect Can Go In Loops
Causality is one of those difficult scientific topics that can easily stray into the realm of philosophy. Science’s relationship with the concept started out simply enough: an event causes another event later in time. That had been the standard understanding of the scientific community up until quantum mechanics was introduced. Then, with the introduction of the famous “spooky action at a distance” that is a side effect of the concept of quantum entanglement, scientists began to question that simple interpretation of causality.
Now, researchers at the Université Libre de Bruxelles (ULB) and the University of Oxford have come up with a theory that further challenges that standard view of causality as a linear progress from cause to effect. In their new theoretical structure, cause and effect can sometimes take place in cycles, with the effect actually causing the cause.
Continue reading “Quantum Theory Proposes That Cause and Effect Can Go In Loops”LIGO Will Squeeze Light To Overcome The Quantum Noise Of Empty Space
When two black holes merge, they release a tremendous amount of energy. When LIGO detected the first black hole merger in 2015, we found that three solar masses worth of energy was released as gravitational waves. But gravitational waves don’t interact strongly with matter. The effects of gravitational waves are so small that you’d need to be extremely close to a merger to feel them. So how can we possibly observe the gravitational waves of merging black holes across millions of light-years?
Continue reading “LIGO Will Squeeze Light To Overcome The Quantum Noise Of Empty Space”French Scientists Claim to Have Created Metallic Hydrogen
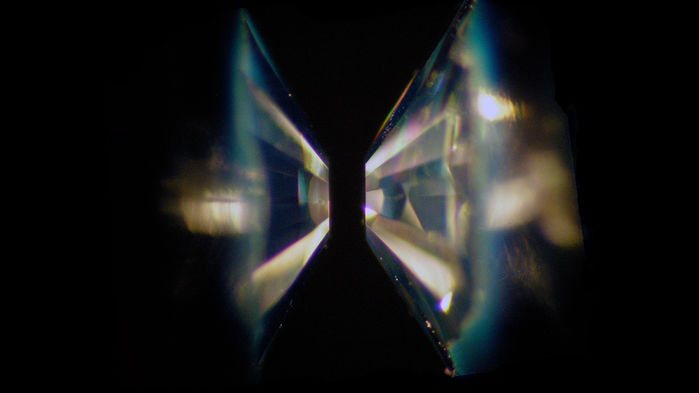
Scientists have long speculated that at the heart of a gas giant, the laws of material physics undergo some radical changes. In these kinds of extreme pressure environments, hydrogen gas is compressed to the point that it actually becomes a metal. For years, scientists have been looking for a way to create metallic hydrogen synthetically because of the endless applications it would offer.
At present, the only known way to do this is to compress hydrogen atoms using a diamond anvil until they change their state. And after decades of attempts (and 80 years since it was first theorized), a team of French scientists may have finally created metallic hydrogen in a laboratory setting. While there is plenty of skepticism, there are many in scientific community who believe this latest claim could be true.
Continue reading “French Scientists Claim to Have Created Metallic Hydrogen”Antimatter Behaves Exactly the Same as Regular Matter in Double Slit Experiments
In 1924, French physicist Louis de Broglie proposed that photons – the subatomic particle that constitutes light – behave as both a particle and a wave. Known as “particle-wave duality”, this property has been tested and shown to apply with other subatomic particles (electrons and neutrons) as well as larger, more complex molecules.
Recently, an experiment conducted by researchers with the QUantum Interferometry and Gravitation with Positrons and LAsers (QUPLAS) collaboration demonstrated that this same property applies to antimatter. This was done using the same kind of interference test (aka. double-slit experiment) that helped scientists to propose particle-wave duality in the first place.
Continue reading “Antimatter Behaves Exactly the Same as Regular Matter in Double Slit Experiments”