Watching the inky-black shadow of a Jovian moon slide across the cloud-tops of Jupiter is an unforgettable sight. Two is always better than one, and as the largest planet in our solar system heads towards opposition on March 8th, so begins the first of two seasons of double shadow transits for 2016. Continue reading “Double Shadow Transit Season for the Jovian Moons Begins”
Obama Administration Proposes Smaller 2017 NASA Budget of $19 Billion with Big Exploration Cuts
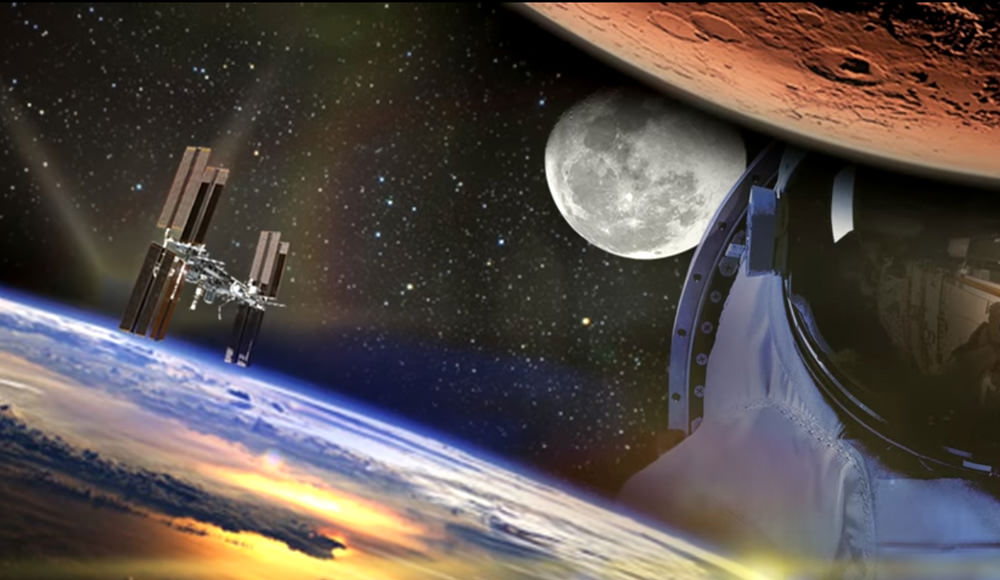
The Obama Administration has announced its new Federal budget and is proposing to cut NASA’s Fiscal Year 2017 Budget to $19 billion by carving away significant funding for deep space exploration, whereas the overall US Federal budget actually increases to over $4.1 trillion.
This 2017 budget request amounts to almost $300 million less than the recently enacted NASA budget for 2016 and specifically stipulates deep funding cuts for deep space exploration programs involving both humans and robots, during President Obama’s final year in office.
The 2017 budget proposal would slash funding to the very programs designed to expand the frontiers of human knowledge and aimed at propelling humans outward to the Red Planet and robots to a Jovian moon that might be conducive to the formation of life.
Absent sufficient and reliable funding to keep NASA’s exploration endeavors on track, further launch delays are almost certainly inevitable – thereby fraying American leadership in space and science.
The administration is specifying big funding cuts to the ongoing development of NASA’s mammoth Space Launch System (SLS) heavy lift rocket and the state of the art Orion deep space crew capsule. They are the essential first ingredients to carry out NASA’s ambitious plans to send astronauts on deep space ‘Journey to Mars’ expeditions during the 2030s.
The overall Exploration Systems Development account for human deep space missions would be slashed about 18 percent from the 2016 funding level; from $4.0 Billion to only $3.3 Billion, or nearly $700 million.
SLS alone is reduced the most by $700 million from $2.0 billion to $1,31 billion, or a whopping 35 percent loss. Orion is reduced from $1.27 billion to $1.12 billion for a loss of some $150 million.
Make no mistake. These programs are already starved for funding and the Obama administration tried to force similar cuts to these programs in 2016, until Congress intervened.
Likewise, the Obama administration is proposing a draconian cut to the proposed robotic mission to Jupiter’s moon Europa that would surely delay the launch by at least another half a decade or more – to the late 2020s.
The Europa mission budget proposal is cut to only $49 million and the launch is postponed until the late 2020s. The mission received $175 million in funding in 2016 – amounting to a 72 percent reduction.
Furthermore there is no funding for a proposed lander and the launch vehicle changes from SLS to a far less powerful EELV – causing a year’s long increased travel time.
In order to maintain an SLS launch in approximately 2022, NASA would require a budget of about $150 million in 2017, said David Radzanowski, NASA’s chief financial officer, during a Feb. 9 teleconference with reporters.
Why is Europa worth exploring? Because Europa likely possesses a subsurface ocean of water and is a prime target in the search for life!
Overall, NASA’s hugely successful Planetary Sciences division suffers a huge and nearly 10 percent cut of $141 million to $1.51 billion – despite undeniably groundbreaking scientific successes this past year at Pluto, Ceres, Mars and more!
Altogether NASA would receive $19.025 billion in FY 2017. This totals $260 million less than the $19.285 billion appropriated in FY 2016, and thus corresponds to a reduction of 1.5 percent.
By contrast, the overall US Federal Budget will increase nearly 5 percent to approximately $4.1 trillion. Simple math demonstrates that NASA is clearly not a high priority for the administration. NASA’s share of the Federal budget comes in at less than half a cent on the dollar.
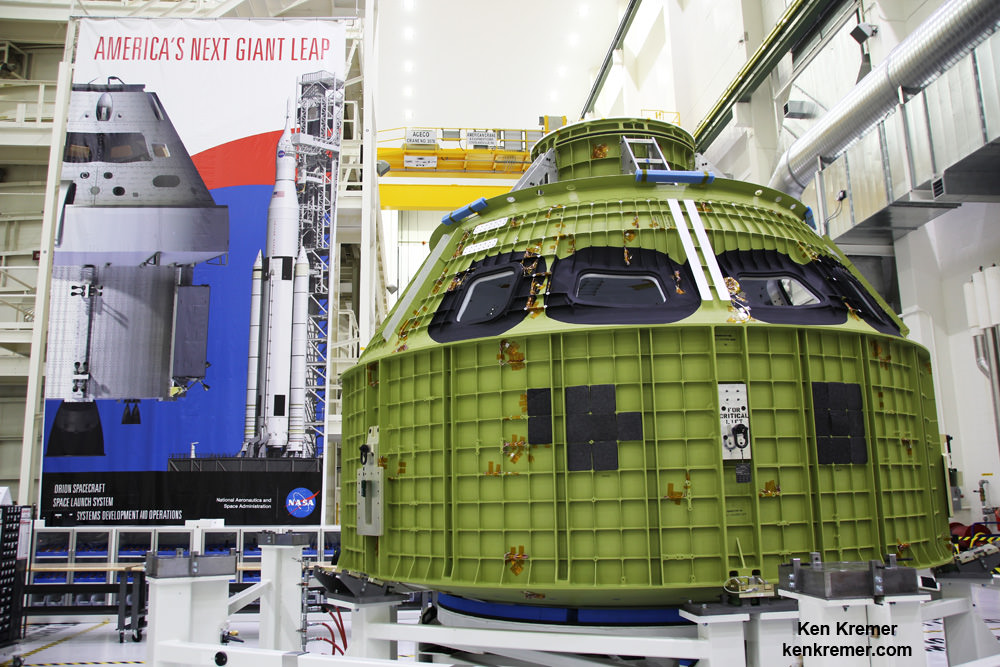
NASA’s Fiscal Year 2017 budget proposal was announced by NASA Administrator Charles Bolden during a televised ‘State of NASA’ address at the agency’s Langley Research Center in Virginia on Feb. 9.
Bolden did not dwell at all on the significant funding reductions for exploration.
“We are hitting our benchmarks with new exploration systems like the Space Launch System rocket and the Orion Crew Vehicle. A new consensus is emerging in the scientific and policy communities around our vision, timetable and plan for sending American astronauts to Mars in the 2030s.”
And he outlined some milestones ahead.
“We’ll continue to make great progress on the Space Launch System – SLS–rocket and we’re preparing for a second series of engine tests,” said Bolden.
“At the Kennedy Space Center, our teams will outfit Orion’s crew module with the spacecraft’s heat-shielding thermal protection systems, avionics and subsystems like electrical power storage, cabin pressure control and flight software –to name just a few.”

NASA plans to launch the first combined SLS/Orion on the uncrewed Exploration Mission-1 (EM-1) in November 2018.
Indeed the Orion EM-1 pressure vessel just arrived at the Kennedy Space Center last week to completely install all the systems required for flight.

The launch date for the first crewed flight on EM-2 was targeted for 2021. But EM-2 is likely to slip to the right to 2023, due to insufficient funding.
Lack of funding will also force NASA to delay development of the far more capable and powerful Exploration Upper Stage (EUS) to propel Orion on deep space missions. It will now not be available for the SLS/EM-2 launch as hoped.
The proposed huge budget cuts to SLS, Orion and Europa are certain to arose the ire of multiple members of Congress and space interest groups, who just successfully fought to increase NASA’s FY 2016 budget for these same programs in the recently passed 2016 omnibus spending bill.
“This administration cannot continue to tout plans to send astronauts to Mars while strangling the programs that will take us there,” said Rep. Lamar Smith (R-Texas), Chairman of the House Science, Space, and Technology Committee, in a statement in response to the president’s budget proposal.
“President Obama’s FY17 budget proposal shrinks our deep space exploration programs by more than $800 million. And the administration once more proposes cuts of more than $100 million to the Planetary Science accounts, which have previously funded missions like this past year’s Pluto flyby.”
“This imbalanced proposal continues to tie our astronauts’ feet to the ground and makes a Mars mission all but impossible. This is not the proposal of an administration that is serious about maintaining America’s leadership in space.”
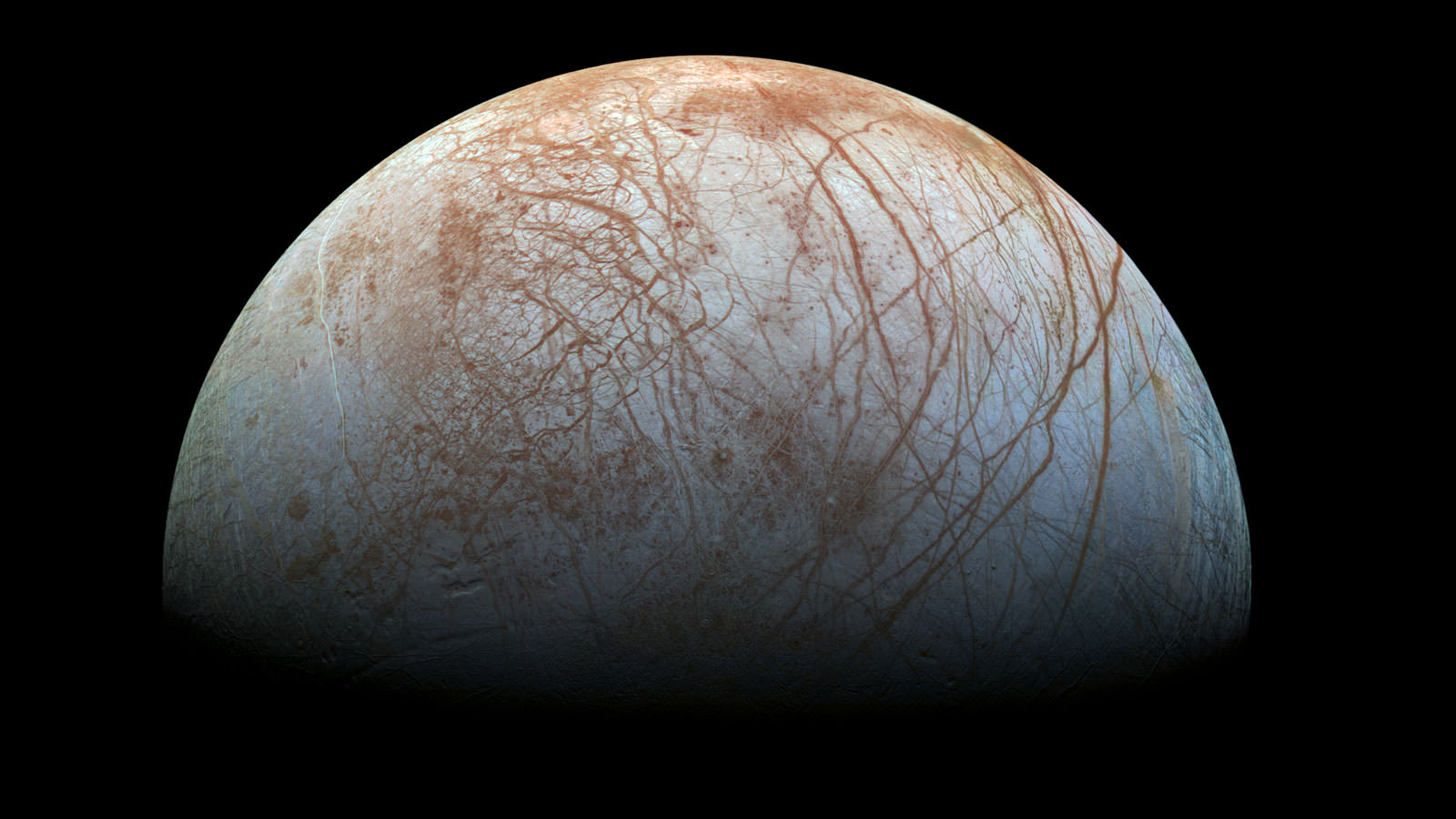
“The Coalition for Deep Space Exploration … had hoped the request would reflect the priorities laid out for NASA in the FY16 Omnibus, for which there was broad support,” said Mary Lynne Dittmar, executive director of the Coalition for Deep Space Exploration, in a statement.
“Unfortunately this was not the case. The Coalition is disappointed with the proposed reduction in funding below the FY16 Omnibus for NASA’s exploration programs. We are deeply concerned about the Administration’s proposed cut to NASA’s human exploration development programs.”
“This proposed budget falls well short of the investment needed to support NASA’s exploration missions, and would have detrimental impacts on cornerstone, game-changing programs such as the super-heavy lift rocket, the Space Launch System (SLS), and the Orion spacecraft – the first spacecraft designed to reach multiple destinations in the human exploration of deep space.”
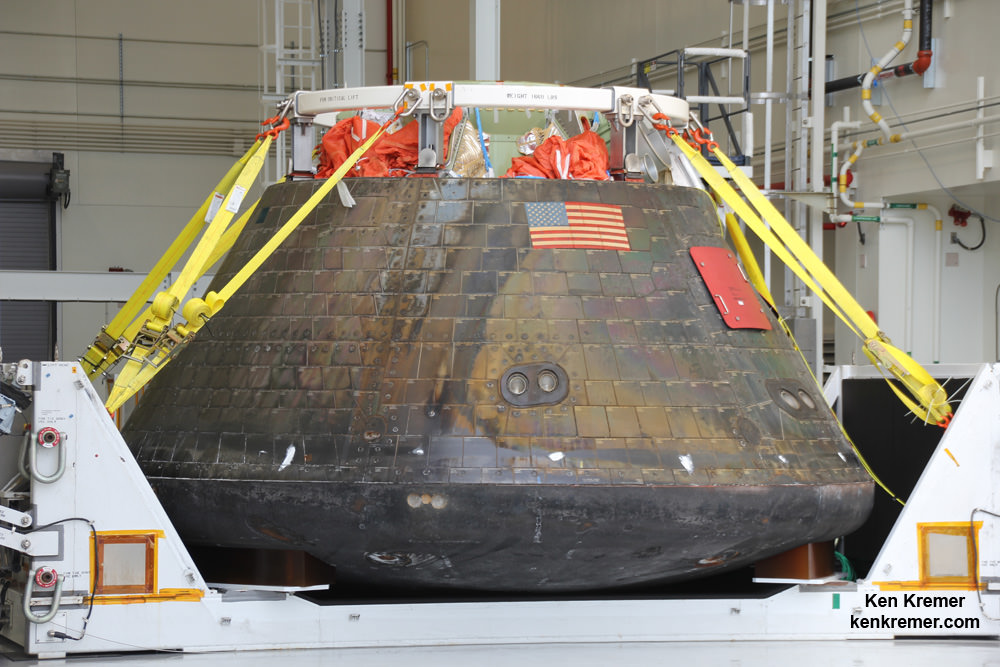
Funding for the James Webb Space Telescope (JWST) was maintained at planned levels to keep it on track for launch in 2018.
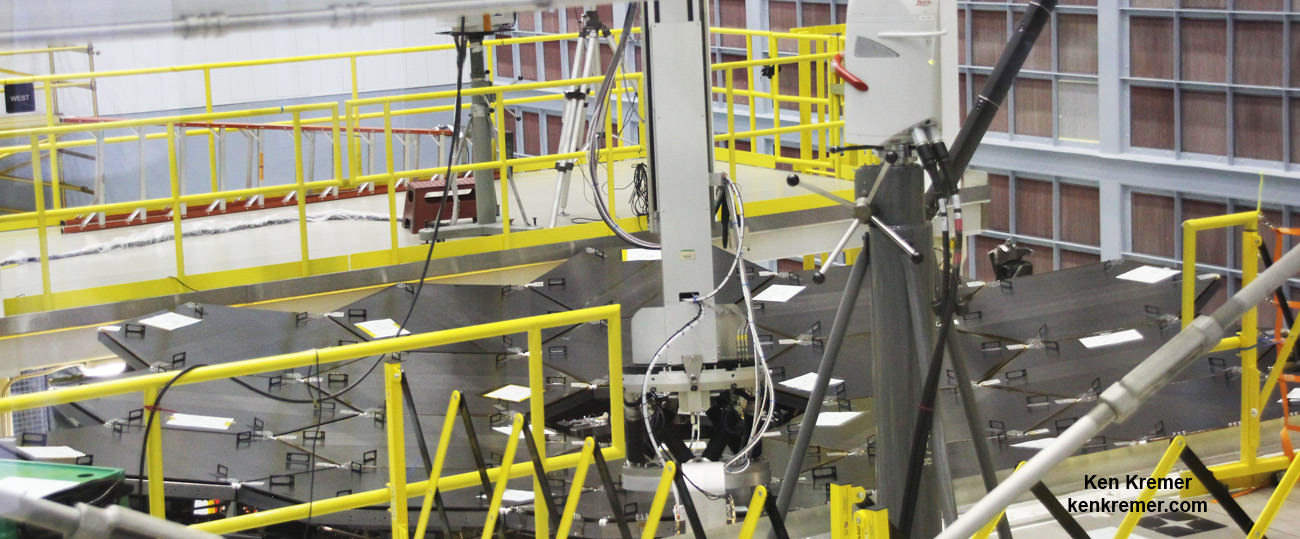
On Dec. 18, 2015, the US Congress passed and the president signed the 2016 omnibus spending bill which funds the US government through the remainder of the 2016 Fiscal Year.
As part of the omnibus bill, NASA’s approved budget amounted to nearly $19.3 Billion. That was an outstanding result and a remarkable turnaround to some long awaited good news from the decidedly negative outlook earlier in 2015.
The 2016 budget represented an increase of some $750 million above the Obama Administration’s proposed NASA budget allocation of $18.5 Billion for Fiscal Year 2016, and an increase of more than $1.2 Billion over the enacted budget for FY 2015.
Under the proposed NASA budget for Fiscal Year 2017, the fictional exploits of ‘The Martian’ will never become reality.
And the hunt for extraterrestrial life on the icy moons of the outer solar system is postponed yet again.
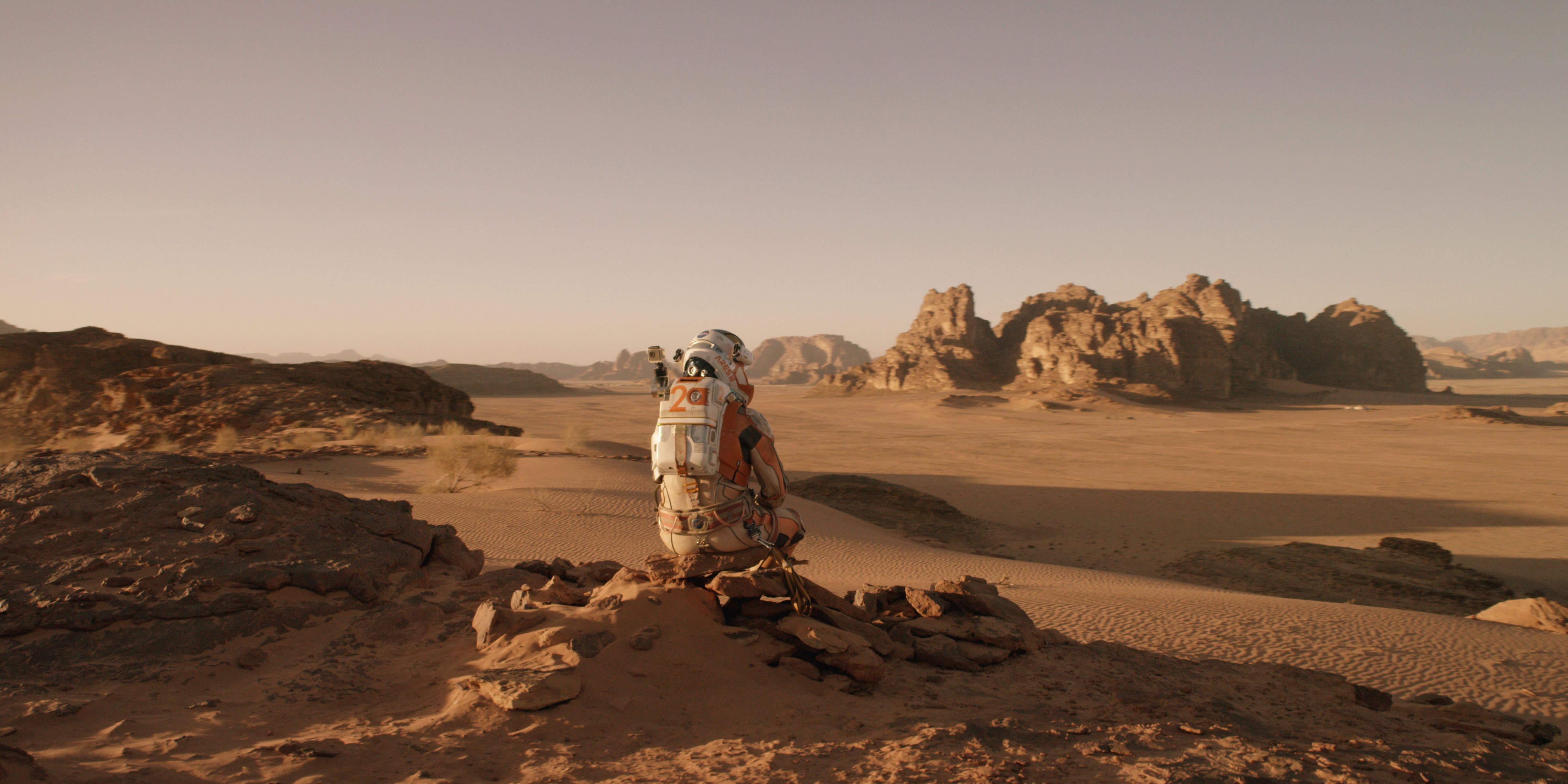
Stay tuned here for Ken’s continuing Earth and Planetary science and human spaceflight news.
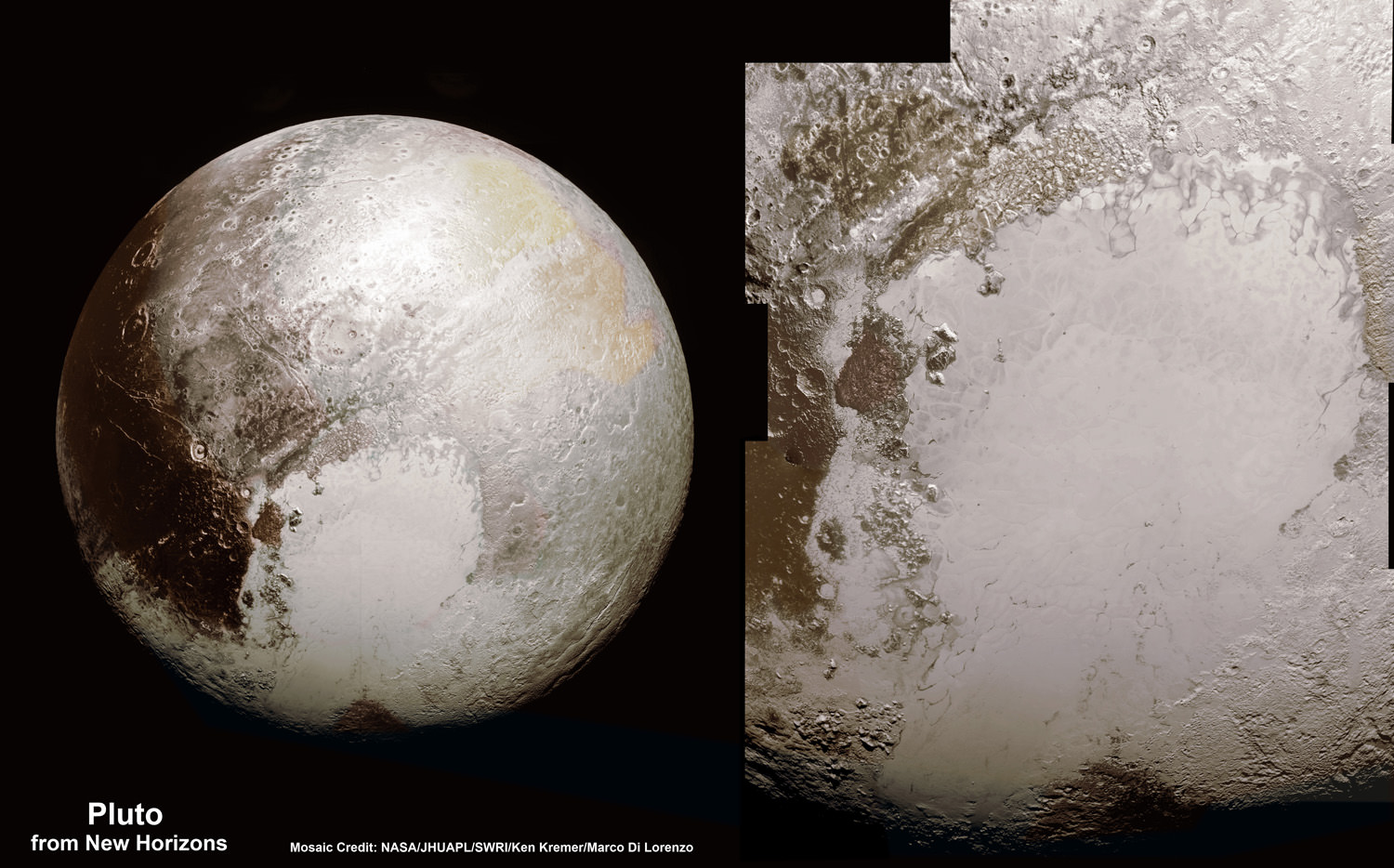
Will We Contaminate Europa?
Europa is probably the best place in the Solar System to go searching for life. But before they’re launched, any spacecraft we send will need to be squeaky clean so don’t contaminate the place with our filthy Earth bacteria.
Continue reading “Will We Contaminate Europa?”
Io, Jupiter’s Volcanic Moon
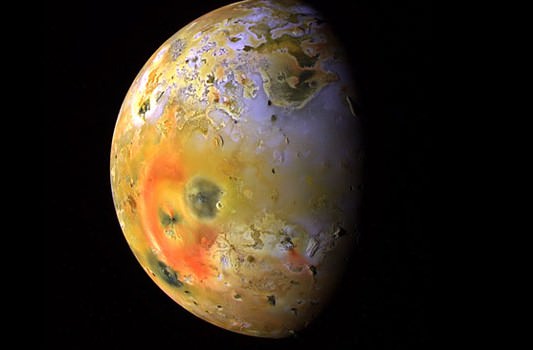
Exploring the Solar System is like peeling an onion. With every layer removed, one finds fresh mysteries to ponder over, each one more confounding than the last. And this is certainly the case when it comes to Jupiter’s system of moons, particularly its four largest – Io, Europa, Ganymede and Callisto. Known as the Galilean Moons, in honor of their founder, these moons possess enough natural wonders to keep scientists busy for centuries.
As Jupiter’s innermost moon, it is also the fourth-largest moon in the Solar System, has the highest density of any known moon, and is the driest known object in the Solar System. It is also one of only four known bodies that experiences active volcanism and – with over 400 active volcanoes – it is the most geologically active body in the Solar System.
Jupiter’s Moon Callisto
With 67 confirmed satellites, Jupiter has the largest system of moons in the Solar System. The greatest of these are the four major moons of Io, Europa, Ganymede and Callisto – otherwise known as the Galilean Moons. Named in honor of their founder, these moons are not only comparable in size to some planets (such as Mercury), they are also some of the few places outside of Earth where liquid water exists, and perhaps even life.
But it is Callisto, the fourth and farthest moon of Jupiter, that may be the most rewarding when it comes to scientific research. In addition to the possibility of a subsurface ocean, this moon is the only Galilean far enough outside of Jupiter’s powerful magnetosphere that it does not experience harmful levels of radiation. This, and the prospect of finding life, make Callisto a prime candidate for future exploration.
Discovery and Naming:
Along with Io, Europa and Ganymede, Callisto was discovered in January of 1610 by Galileo Galilei using a telescope of his own design. Like all the Galilean Moons, it takes its name from one of Zeus’ lovers in classic Greek mythology. Callisto was a nymph (or the daughter of Lycaon) who was associated with the goddess of the hunt, Artemis.
The name was suggested by German astronomer Simon Marius, apparently at the behest of Johannes Kepler. However, Galileo initially refused to use them, and the moons named in his honor were designed as Jupiter I through IV, based on their proximity to their parent planet. Being the farthest planet from Jupiter, Callisto was known as Jupiter IV until the 20th century, by which time, the names suggested by Marius were adopted.
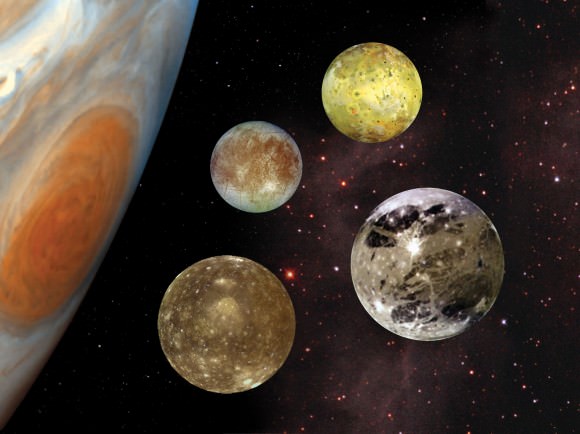
Size, Mass and Orbit:
With a mean radius of 2410.3 ± 1.5 km (0.378 Earths) and a mass of 1.0759 × 1023 kg (0.018 Earths), Callisto is the second largest Jupiter’s moons (after Ganymede) and the third largest satellite in the solar system. Much like Ganymede, it is comparable in size to Mercury – being 99% as large – but due to its mixed composition, it has less than one-third of Mercury mass.
Callisto orbits Jupiter at an average distance (semi-major axis) of 1,882,700 km. It has a very minor eccentricity (0.0074) and ranges in distance from 1,869,000 km at periapsis to 1,897,000 km at apoapsis. This distance, which is far greater than Ganymede’s, means that Callisto does not take part in the mean-motion resonance that Io, Europa and Ganymede do.
Much like the other Galileans, Callisto’s rotation is synchronous with its orbit. This means that it takes the same amount of time (16.689 days) for Callisto to complete a single orbit of Jupiter and a single rotation on its axis. Its orbit is very slightly eccentric and inclined to the Jovian equator, with the eccentricity and inclination changing over the course of centuries due to solar and planetary gravitational perturbations.
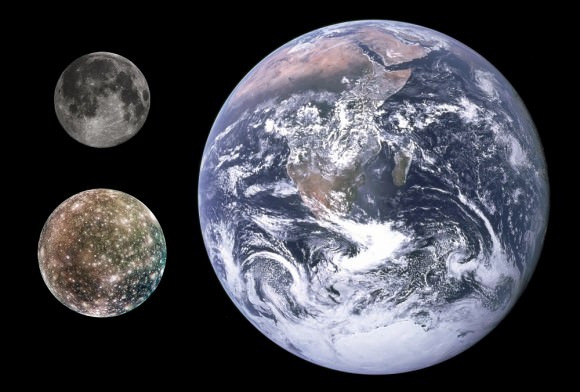
Unlike the other Galileans, Callisto’s distant orbit means that it has never experienced much in the way of tidal-heating, which has had a profound impact on its internal structure and evolution. Its distance from Jupiter also means that the charged particles from Jupiter’s magnetosphere have had a very minor influence on its surface.
Composition and Surface Features:
The average density of Callisto, at 1.83 g/cm3, suggests a composition of approximately equal parts of rocky material and water ice, with some additional volatile ices such as ammonia. Ice is believed to constitute 49-55% of the moon, with the rock component likely made up of chondrites, silicates and iron oxide.
Callisto’s surface composition is thought to be similar to its composition as a whole, with water ice constituting 25-50% of its overall mass. High-resolution, near-infrared and UV spectra imaging have revealed the presence of various non-ice materials, such as magnesium and iron-bearing hydrated silicates, carbon dioxide, sulfur dioxide, and possibly ammonia and various organic compounds.
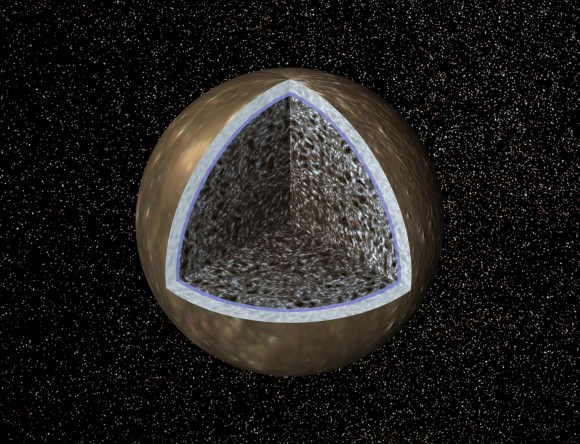
Beneath the surface is an icy lithosphere that is between 80-150 m thick. A salty ocean 50–200 km deep is believed to exist beneath this, thanks to the presence of radioactive elements and the possible existence of ammonia. Evidence of this ocean include Jupiter’s magnetic field, which shows no signs of penetrating Callisto’s surface. This suggests a layer of highly conductive fluid that is at least 10 km in depth. However, if this water contains ammonia, which is more likely, than it could be up to 250-300 km.
Beneath this hypothetical ocean, Callisto’s interior appears to be composed of compressed rocks and ices, with the amount of rock increasing with depth. This means, in effect, that Callisto is only partially differentiated, with a small silicate core no larger than 600 km (and a density of 3.1-3.6 g/cm³) surrounded by a mix of ice and rock.
Spectral data has also indicated that Callisto’s surface is extremely heterogeneous at the small scale. Basically, the surface consists of small, bright patches of pure water ice, intermixed with patches of a rock–ice mixture, and extended dark areas made of a non-ice material.
Compared to the other Galilean Moons, Callisto’s surface is quite dark, with a surface albedo of about 20%. Another difference is the nature of its asymmetric appearance. Whereas with the other Galileans, the leading hemisphere is lighter than the trailing one, with Callisto the opposite is true.

An immediately obvious feature about Callisto’s surface is the ancient and heavily cratered nature of it. In fact, the surface is the most cratered in the Solar System and is almost entirely saturated by craters, with newer ones having formed over older ones. What’s more, impact craters and their associated structures are the only large features on the surface. There are no mountains, volcanoes or other endogenic tectonic features.
Callisto’s impact craters range in size from 0.1 km to over 100 km, not counting the multi-ring structures. Small craters, with diameters less than 5 km, have simple bowl or flat-floored shapes, whereas those that measure 5–40 km usually have a central peak.
Larger impact features, with diameters that range from 25–100 km have central pits instead of peaks. Those with diameters over 60 km can have central domes, which are thought to result from central tectonic uplift after an impact.
The largest impact features on Callisto’s surface are multi-ring basins, which probably originated as a result of post-impact concentric fracturing which took place over a patch of lithosphere that overlay a section of soft or liquid material (possibly a patch of the interior ocean). The largest of these are Valhalla and Asgard, whose central, bright regions measure 600 and 1600 km in diameter (respectively) with rings extending farther outwards.
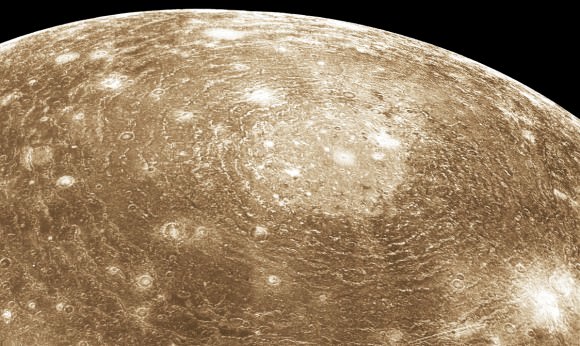
The relative ages of the different surface units on Callisto can be determined from the density of impact craters on them – the older the surface, the denser the crater population. Based on theoretical considerations, the cratered plains are thought to be ~4.5 billion years old, dating back almost to the formation of the Solar System.
The ages of multi-ring structures and impact craters depend on chosen background cratering rates, and are estimated by different researchers to vary between 1 and 4 billion years of age.
Atmosphere:
Callisto has a very tenuous atmosphere composed of carbon dioxide which has an estimated surface pressure of 7.5 × 10-¹² bar (0.75 micro Pascals) and a particle density of 4 × 108 cm-3. Because such a thin atmosphere would be lost in only about 4 days, it must be constantly replenished, possibly by slow sublimation of carbon dioxide ice from Callisto’s icy crust.
While it has not been directly detected, it is believed that molecular oxygen exists in concentrations 10-100 times greater than CO². This is evidenced by the high electron density of the planet’s ionosphere, which cannot be explained by the photoionization of carbon dioxide alone. However, condensed oxygen has been detected on the surface of Callisto, trapped within its icy crust.
Habitability:
Much like Europa and Ganymede, and Saturn’s moons of Enceladus, Mimas, Dione, Titan, the possible existence of a subsurface ocean on Callisto has led many scientists to speculate about the possibility of life. This is particularly likely if the interior ocean is made up of salt-water, since halophiles (which thrive in high salt concentrations) could live there.
In addition, the possibility of extra-terrestrial microbial life has also been raised with respect to Callisto. However, the environmental conditions necessary for life to appear (which include the presence of sufficient heat due to tidal flexing) are more likely on Europa and Ganymede. The main difference is the lack of contact between the rocky material and the interior ocean, as well as the lower heat flux in Callisto’s interior.
In essence, while Callisto possesses the necessary pre-biotic chemistry to host life, it lacks the necessary energy. Because of this, the most likely candidate for the existence of extra-terrestrial life in Jupiter’s system of moons remains Europa.
Exploration:
The first exploration missions to Callisto were the Pioneer 10 and 11 spacecrafts, which conducted flybys of the Galilean moon in 1973 and 1974, respectively, But these missions provided little additional information beyond what had already learned through Earth-based observations. In contrast, the Voyager 1 and 2 spacecraft, which conducted flybys of the moon in 1979, managed to image more than half the surface and precisely measured Callisto’s temperature, mass and shape.
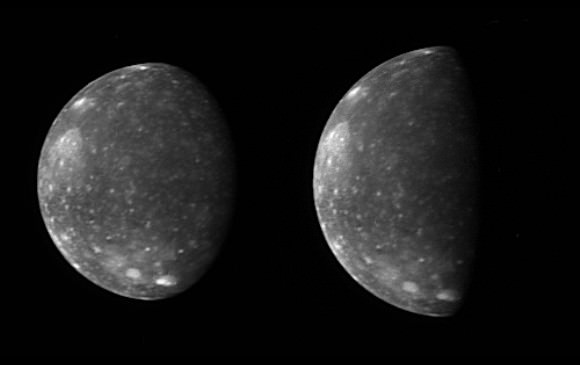
Further exploration took place between 1994 and 2003, when the Galileo spacecraft performed eight close flybys with Callisto. The orbiter completed the global imaging of the surface and delivered a number of pictures with a resolution as high as 15 meters. In 2000, while en route to Saturn, the Cassini spacecraft acquired high-quality infrared spectra of the Galilean satellites, including Callisto.
In February–March 2007, while en route to Pluto, the New Horizons probe obtained new images and spectra of Callisto. Using its Linear Etalon Imaging Spectral Array (LEISA) instrument, the probe was able to reveal how lighting and viewing conditions affect infrared spectrum readings of its surface water ice.
The next planned mission to the Jovian system is the European Space Agency’s Jupiter Icy Moon Explorer (JUICE), due to launch in 2022. Ostensibly geared towards exploring Europa and Ganymede, the mission profile also includes several close flybys of Callisto.
Colonization:
Compared to the other Galileans, Callisto presents numerous advantages as far as colonization is concerned. Much like the others, the moon has an abundant supply of water in the form of surface ice (but also possibly liquid water beneath the surface). But unlike the others, Callisto’s distance from Jupiter means that colonists would have far less to worry about in terms of radiation.
In 2003, NASA conducted a conceptual study called Human Outer Planets Exploration (HOPE) regarding the future human exploration of the outer Solar System. The target chosen to consider in detail was Callisto, for the purposes of investigating the possible existence of life forms embedded in the ice crust on this moon and on Europa.
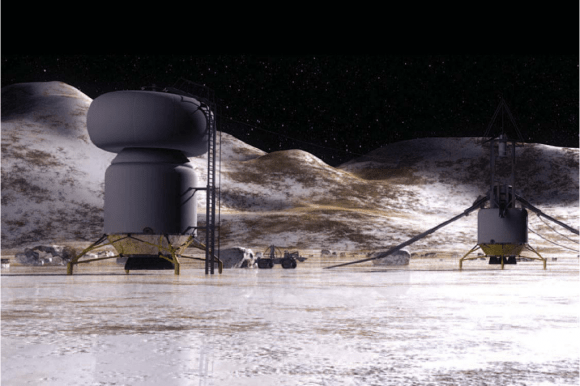
The study proposed a possible surface base on Callisto where a crew could “teleoperate a Europa submarine and excavate Callisto surface samples near the impact site”. In addition, this base could extract water from Callisto’s ample supply of water ices to produce rocket propellant for further exploration of the Solar System.
The advantages of a base on Callisto include low radiation (due to its distance from Jupiter) and geological stability. Such a base could facilitate exploration on other Galilean Moons, and be an ideal location for a Jovian system way station, servicing spacecraft heading farther into the outer Solar System – which would likely take the form of craft using a gravity assist from a close flyby of Jupiter.
Reports filed by NASA’s Glenn Research Center and Langley Research Center – in December and February of 2003, respectively – both outlined possible manned missions to Callisto, as envisioned by HOPE. According to these reports, a mission that would likely involve a ship using a Mangetoplasmadynamic (MPD) or Nuclear-Electric Propulsion (NEP) drive system, and equipped to generate artificial gravity, could be mounted in the 2040s.
So while Callisto may not be the best target in the search for extra-terrestrial life, it may be the most hospitable of Jupiter’s moons for human life. In either case, any future missions to Jupiter will likely include a stopovers to Callisto, with the intent of investigating both of these possibilities.
We have many great articles on Callisto, Jupiter, and its system of moons here at Universe Today. Here’s one about how impacts effected Callisto’s interior, And here is one on all of the Galilean Moons.
For more information, check out NASA’s Solar System Exploration page on Callisto.
Astronomy Cast offers has a good episode on the subject, titled Episode 57: Jupiter’s Moons.
Jupiter’s Moon Ganymede

In 1610, Galileo Galilei looked up at the night sky through a telescope of his own design. Spotting Jupiter, he noted the presence of several “luminous objects” surrounding it, which he initially took for stars. In time, he would notice that these “stars” were orbiting the planet, and realized that they were in fact Jupiter’s moons – which would come to be named Io, Europa, Ganymede and Callisto.
Of these, Ganymede is the largest, and boasts many fascinating characteristics. In addition to being the largest moon in the Solar System, it is also larger than even the planet Mercury. It is the only satellite in the Solar System known to possess a magnetosphere, has a thin oxygen atmosphere, and (much like its fellow-moons, Europa and Callisto) is believed to have an interior ocean.
Continue reading “Jupiter’s Moon Ganymede”
Jupiter’s Moon Europa
Jupiter‘s four largest moons – aka. the Galilean Moons, consisting of Io, Europa, Ganymede, and Callisto – are nothing if not fascinating. Ever since their discovery over four centuries ago, these moons have been a source of many great discoveries. These include the possibility of internal oceans, the presence of atmospheres, volcanic activity, a magnetosphere (Ganymede), and the possibility of having more water than Earth.
But arguably, the most fascinating of the Galilean Moons is Europa: the sixth closest moon to Jupiter, the smallest of the four, and the sixth-largest moon in the Solar System. In addition to having an icy surface and a possible warm-water interior, this moon is considered to be one of the most likely places for finding life beyond Earth.
Continue reading “Jupiter’s Moon Europa”The Planet Jupiter
Ever since the invention of the telescope four hundred years ago, astronomers have been fascinated by the gas giant known as Jupiter. Between its constant, swirling clouds, its many, many moons, and its Giant Red Spot, there are many things about this planet that are both delightful and fascinating.
But perhaps the most impressive feature about Jupiter is its sheer size. In terms of mass, volume, and surface area, Jupiter is the biggest planet in our Solar System by a wide margin. And since people have been aware of its existence for thousands of years, it has played an active role in the cosmological systems many cultures. But just what makes Jupiter so massive, and what else do we know about it?
Size, Mass and Orbit:
Jupiter’s mass, volume, surface area and mean circumference are 1.8981 x 1027 kg, 1.43128 x 1015 km3, 6.1419 x 1010 km2, and 4.39264 x 105 km respectively. To put that in perspective, Jupiter diameter is roughly 11 times that of Earth, and 2.5 the mass of all the other planets in the Solar System combined.
But, being a gas giant, it has a relatively low density – 1.326 g/cm3 – which is less than one quarter of Earth’s. This means that while Jupiter’s volume is equivalent to about 1,321 Earths, it is only 318 times as massive. The low density is one way scientists are able to determine that it is made mostly of gases, though the debate still rages on what exists at its core (see below).
Jupiter orbits the Sun at an average distance (semi-major axis) of 778,299,000 km (5.2 AU), ranging from 740,550,000 km (4.95 AU) at perihelion and 816,040,000 km (5.455 AU) at aphelion. At this distance, Jupiter takes 11.8618 Earth years to complete a single orbit of the Sun. In other words, a single Jovian year lasts the equivalent of 4,332.59 Earth days.
However, Jupiter’s rotation is the fastest of all the Solar System’s planets, completing a rotation on its axis in slightly less than ten hours (9 hours, 55 minutes and 30 seconds to be exact. Therefore, a single Jovian year lasts 10,475.8 Jovian solar days. This orbital period is two-fifths that of Saturn, which means that the two largest planets in our Solar System form a 5:2 orbital resonance.
Structure and Composition:
Jupiter is composed primarily of gaseous and liquid matter. It is the largest of the gas giants, and like them, is divided between a gaseous outer atmosphere and an interior that is made up of denser materials. It’s upper atmosphere is composed of about 88–92% hydrogen and 8–12% helium by percent volume of gas molecules, and approx. 75% hydrogen and 24% helium by mass, with the remaining one percent consisting of other elements.
The atmosphere contains trace amounts of methane, water vapor, ammonia, and silicon-based compounds as well as trace amounts of benzene and other hydrocarbons. There are also traces of carbon, ethane, hydrogen sulfide, neon, oxygen, phosphine, and sulfur. Crystals of frozen ammonia have also been observed in the outermost layer of the atmosphere.
The interior contains denser materials, such that the distribution is roughly 71% hydrogen, 24% helium and 5% other elements by mass. It is believed that Jupiter’s core is a dense mix of elements – a surrounding layer of liquid metallic hydrogen with some helium, and an outer layer predominantly of molecular hydrogen. The core has also been described as rocky, but this remains unknown as well.
In 1997, the existence of the core was suggested by gravitational measurements, indicating a mass of from 12 to 45 times the Earth’s mass, or roughly 4%–14% of the total mass of Jupiter. The presence of a core is also supported by models of planetary formation that indicate how a rocky or icy core would have been necessary at some point in the planet’s history in order to collect all of its hydrogen and helium from the protosolar nebula.
However, it is possible that this core has since shrunk due to convection currents of hot, liquid, metallic hydrogen mixing with the molten core. This core may even be absent now, but a detailed analysis is needed before this can be confirmed. The Juno mission, which launched in August 2011 (see below), is expected to provide some insight into these questions, and thereby make progress on the problem of the core.
The temperature and pressure inside Jupiter increase steadily toward the core. At the “surface”, the pressure and temperature are believed to be 10 bars and 340 K (67 °C, 152 °F). At the “phase transition” region, where hydrogen becomes metallic, it is believed the temperature is 10,000 K (9,700 °C; 17,500 °F) and the pressure is 200 GPa. The temperature at the core boundary is estimated to be 36,000 K (35,700 °C; 64,300 °F) and the interior pressure at roughly 3,000–4,500 GPa.
Jupiter’s Moons:
The Jovian system currently includes 67 known moons. The four largest are known as the Galilean Moons, which are named after their discoverer, Galileo Galilei. They include: Io, the most volcanically active body in our Solar System; Europa, which is suspected of having a massive subsurface ocean; Ganymede, the largest moon in our Solar System; and Callisto, which is also thought to have a subsurface ocean and features some of the oldest surface material in the Solar System.
Then there’s the Inner Group (or Amalthea group), which is made up of four small moons that have diameters of less than 200 km, orbit at radii less than 200,000 km, and have orbital inclinations of less than half a degree. This groups includes the moons of Metis, Adrastea, Amalthea, and Thebe. Along with a number of as-yet-unseen inner moonlets, these moons replenish and maintain Jupiter’s faint ring system.
Jupiter also has an array of Irregular Satellites, which are substantially smaller and have more distant and eccentric orbits than the others. These moons are broken down into families that have similarities in orbit and composition, and are believed to be largely the result of collisions from large objects that were captured by Jupiter’s gravity.
Atmosphere and Storms:
Much like Earth, Jupiter experiences auroras near its northern and southern poles. But on Jupiter, the auroral activity is much more intense and rarely ever stops. The intense radiation, Jupiter’s magnetic field, and the abundance of material from Io’s volcanoes that react with Jupiter’s ionosphere create a light show that is truly spectacular.
Jupiter also experiences violent weather patterns. Wind speeds of 100 m/s (360 km/h) are common in zonal jets, and can reach as high as 620 kph (385 mph). Storms form within hours and can become thousands of km in diameter overnight. One storm, the Great Red Spot, has been raging since at least the late 1600s. The storm has been shrinking and expanding throughout its history; but in 2012, it was suggested that the Giant Red Spot might eventually disappear.
Jupiter is perpetually covered with clouds composed of ammonia crystals and possibly ammonium hydrosulfide. These clouds are located in the tropopause and are arranged into bands of different latitudes, known as “tropical regions”. The cloud layer is only about 50 km (31 mi) deep, and consists of at least two decks of clouds: a thick lower deck and a thin clearer region.
There may also be a thin layer of water clouds underlying the ammonia layer, as evidenced by flashes of lightning detected in the atmosphere of Jupiter, which would be caused by the water’s polarity creating the charge separation needed for lightning. Observations of these electrical discharges indicate that they can be up to a thousand times as powerful as those observed here on the Earth.
Historical Observations of the Planet:
As a planet that can be observed with the naked eye, humans have known about the existence of Jupiter for thousands of years. It has therefore played a vital role in the mythological and astrological systems of many cultures. The first recorded mentions of it date back to the Babylon Empire of the 7th and 8th centuries BCE.
In the 2nd century, the Greco-Egyptian astronomer Ptolemy constructed his famous geocentric planetary model that contained deferents and epicycles to explain the orbit of Jupiter relative to the Earth (i.e. retrograde motion). In his work, the Almagest, he ascribed an orbital period of 4332.38 days to Jupiter (11.86 years).
In 499, Aryabhata – a mathematician-astronomer from the classical age of India – also used a geocentric model to estimate Jupiter’s period as 4332.2722 days, or 11.86 years. It has also been ventured that the Chinese astronomer Gan De discovered Jupiter’s moons in 362 BCE without the use of instruments. If true, it would mean that Galileo was not the first to discovery the Jovian moons two millennia later.
In 1610, Galileo Galilei was the first astronomer to use a telescope to observe the planets. In the course of his examinations of the outer Solar System, he discovered the four largest moons of Jupiter (now known as the Galilean Moons). The discovery of moons other than Earth’s was a major point in favor of Copernicus’ heliocentric theory of the motions of the planets.
During the 1660s, Cassini used a new telescope to discover Jupiter’s spots and colorful bands and observed that the planet appeared to be an oblate spheroid. By 1690, he was also able to estimate the rotation period of the planet and noticed that the atmosphere undergoes differential rotation. In 1831, German astronomer Heinrich Schwabe produced the earliest known drawing to show details of the Great Red Spot.
In 1892, E. E. Barnard observed a fifth satellite of Jupiter using the refractor telescope at the Lick Observatory in California. This relatively small object was later named Amalthea, and would be the last planetary moon to be discovered directly by visual observation.
In 1932, Rupert Wildt identified absorption bands of ammonia and methane in the spectra of Jupiter; and by 1938, three long-lived anticyclonic features termed “white ovals” were observed. For several decades, they remained as separate features in the atmosphere, sometimes approaching each other but never merging. Finally, two of the ovals merged in 1998, then absorbed the third in 2000, becoming Oval BA.
Beginning in the 1950s, radiotelescopic research of Jupiter began. This was due to astronomers Bernard Burke and Kenneth Franklin’s detection of radio signals coming from Jupiter in 1955. These bursts of radio waves, which corresponded to the rotation of the planet, allowed Burke and Franklin to refine estimates of the planet’s rotation rate.
Over time, scientists discovered that there were three forms of radio signals transmitted from Jupiter – decametric radio bursts, decimetric radio emissions, and thermal radiation. Decametric bursts vary with the rotation of Jupiter, and are influenced by the interaction of Io with Jupiter’s magnetic field.
Decimetric radio emissions – which originate from a torus-shaped belt around Jupiter’s equator – are caused by cyclotronic radiation from electrons that are accelerated in Jupiter’s magnetic field. Meanwhile, thermal radiation is produced by heat in the atmosphere of Jupiter. Visualizations of Jupiter using radiotelescopes have allowed astronomers to learn much about its atmosphere, thermal properties and behavior.
Exploration:
Since 1973, a number of automated spacecraft have been sent to the Jovian system and performed planetary flybys that brought them within range of the planet. The most notable of these was Pioneer 10, the first spacecraft to get close enough to send back photographs of Jupiter and its moons. Between this mission and Pioneer 11, astronomers learned a great deal about the properties and phenomena of this gas giant.
For example, they discovered that the radiation fields near the planet were much stronger than expected. The trajectories of these spacecraft were also used to refine the mass estimates of the Jovian system, and radio occultations by the planet resulted in better measurements of Jupiter’s diameter and the amount of polar flattening.
Six years later, the Voyager missions began, which vastly improved the understanding of the Galilean moons and discovered Jupiter’s rings. They also confirmed that the Great Red Spot was anticyclonic, that its hue had changed sine the Pioneer missions – turning from orange to dark brown – and spotted lightning on its dark side. Observations were also made of Io, which showed a torus of ionized atoms along its orbital path and volcanoes on its surface.
On December 7th, 1995, the Galileo orbiter became the first probe to establish orbit around Jupiter, where it would remain for seven years. During its mission, it conducted multiple flybys of all the Galilean moons and Amalthea and deployed an probe into the atmosphere. It was also in the perfect position to witness the impact of Comet Shoemaker–Levy 9 as it approached Jupiter in 1994.
On September 21st, 2003, Galileo was deliberately steered into the planet and crashed in its atmosphere at a speed of 50 km/s, mainly to avoid crashing and causing any possible contamination to Europa – a moon which is believed to harbor life.
Data gathered by both the probe and orbiter revealed that hydrogen composes up to 90% of Jupiter’s atmosphere. The temperatures data recorded was more than 300 °C (570 °F) and the wind speed measured more than 644 kmph (400 mph) before the probe vaporized.
In 2000, the Cassini probe (while en route to Saturn) flew by Jupiter and provided some of the highest-resolution images ever taken of the planet. While en route to Pluto, the New Horizons space probe flew by Jupiter and measured the plasma output from Io’s volcanoes, studied all four Galileo moons in detail, and also conducting long-distance observations of Himalia and Elara.
NASA’s Juno mission, which launched in August 2011, achieved orbit around the Jovian planet on July 4th, 2016. The purpose of this mission to study Jupiter’s interior, its atmosphere, its magnetosphere and gravitational field, ultimately for the purpose of determining the history of the planet’s formation (which will shed light on the formation of the Solar System).
As the probe entered its polar elliptical orbit on July 4th after completing a 35-minute-long firing of the main engine, known as Jupiter Orbital Insertion (or JOI). As the probe approached Jupiter from above its north pole, it was afforded a view of the Jovian system, which it took a final picture of before commencing JOI.
On July 10th, the Juno probe transmitted its first imagery from orbit after powering back up its suite of scientific instruments. The images were taken when the spacecraft was 4.3 million km (2.7 million mi) from Jupiter and on the outbound leg of its initial 53.5-day capture orbit. The color image shows atmospheric features on Jupiter, including the famous Great Red Spot, and three of the massive planet’s four largest moons – Io, Europa and Ganymede, from left to right in the image.
The next planned mission to the Jovian system will be performed by the European Space Agency’s Jupiter Icy Moon Explorer (JUICE), due to launch in 2022, followed by NASA’s Europa Clipper mission in 2025.
Exoplanets:
The discovery of exoplanets has revealed that planets can get even bigger than Jupiter. In fact, the number of “Super Jupiters” observed by the Kepler space probe (as well as ground-based telescopes) in the past few years has been staggering. In fact, as of 2015, more than 300 such planets have been identified.
Notable examples include PSR B1620-26 b (Methuselah), which was the first super-Jupiter to be observed (in 2003). At 12.7 billion years of age, it is also the third oldest known planet in the universe. There’s also HD 80606 b (Niobe), which has the most eccentric orbit of any known planet, and 2M1207b (Lerna), which orbits the brown dwarf Fomalhaut b (Illion).
Here’s an interesting fact. Scientist theorize that a gas gain could get 15 times the size of Jupiter before it began deuterium fusion, making it a brown dwarf star. Good thing too, since the last thing the Solar System needs is for Jupiter to go nova!
Jupiter was appropriately named by the ancient Romans, who chose to name after the king of the Gods (also known as Jove). The more we have come to know and understand about this most-massive of Solar planets, the more deserving of this name it appears.
We have many interesting articles on Jupiter here at Universe Today. Here are some articles on the color and gravity of Jupiter, how it got its name, and how it shaped our Solar System.
Got questions about Jupiter’s greater mysteries? Then here’s Does Jupiter Have a Solid Core?, Could Jupiter Become a Star?, Could We Live on Jupiter?, and Could We Terraform Jupiter?
We have recorded a whole series of podcasts about the Solar System at Astronomy Cast.
What About a Mission to Europa?
Europa’s water exists in a layer around the planet, encased in a layer of ice. Could there be life down there?
Hooray! Welcome to the 200th official episode of the Guide To Space!
First off, thank you. Thank you for watching, liking, sharing, subscribing and being a patron of our show. Yes, you. Thank you.
So to celebrate, a few weeks ago we invited the members of the Weekly Space Hangout Crew Google+ Community to suggest topics for episodes, and the winner would receive a precious iron-nickel meteorite. Congratulations Andres Munoz, this meteorite is for you.
This episode, chosen by Andres, is for everyone.
The search for life in the Solar System is about the hunt for water. Wherever we find liquid water on Earth, we find life. I’m talking everywhere. In the most briny, salty pools in Antarctica, in the hottest hot springs in Yellowstone, under glaciers, and kilometers deep underground.
So we go searching for liquid water in the Solar System.
You might be surprised to learn that Jupiter’s moon Europa has the most water in the entire Solar System. If you took all the water on Earth, collected it into a big sphere, it would measure almost 1,400 kilometers across.
Europa’s water would measure nearly 1,800 kilometers.All that water exists in a layer around Europa, encased in a layer of ice. How thick? We don’t know.
Is there life down there? We don’t know.You can say there might be, and it wouldn’t be untrue. However, if you say there isn’t, that’s way less interesting for clickbait purposes. Whenever we don’t know the answers to fundamental and intriguing questions like that, it’s time to send a mission.
Good news! An actual mission to Europa is in the works right now. In 2015, NASA approved the development of an orbiter mission to Europa. If all goes well, and nothing gets cancelled…
And nothing will get cancelled, right? Right? I heard Firefly. Which one of you said Firefly?!?
According to the plan, a spacecraft will launch in the 2020s, carrying 9 instruments to Europa. Most will be familiar cameras, mass spectrometers, and the like, to study the surface of Europa to a high level of resolution. Over the course of 45 flybys, the spacecraft will get down as close as 25 kilometers and capture it with incredible resolution.
Perhaps the most exciting, and controversial instrument on board the new Europa Orbiter mission will be its ice-penetrating radar. Mission planners battled over installing a radar this sophisticated, as it will be an enormous drain on the orbiter’s power.
This for us is incredibly exciting. It will allow the spacecraft to map out the depth and thickness of Europa’s icy exterior. Is it thick or thin? Are there pockets of water trapped just below the surface, or is it tough shell that goes on for dozens of kilometers?
The worst case scenario is that the shell goes thicker than the radar can reach, and we won’t even know how far it goes.
Whatever happens, the Europa orbiter will be a boon to science, answer outstanding questions about the moon and the chances of finding life there.
We’re just getting started. What we really want to send is a lander. Because of the intense radiation from Jupiter, the Sun, and space itself, the surface of the ice on Europa would be sterilized. But dig down a few centimeters and you might find life that’s protected from the radiation.
A future Europa lander might be equipped with a heated drill attached to a tether. The lander would be have with a heat-generated radioisotope thermoelectric generator, like most of NASA’s big, outer Solar System spacecraft.
But in addition to using it for electricity, it’ll use the raw heat to help a tethered drill to grind through the ice a few meters and sample what’s down there.
Drilling more than a few meters is probably the stuff of science fiction. Russian scientists in Antarctica drilled for almost two decades to get through 4,000 meters of ice above Lake Vostok. Imagine trying to get through 100 kilometers of the stuff, on a distant world, with a robot.
But, since I’ve talked about moving the Sun, and terraforming the Moon, maybe I shouldn’t put any bounds on my imagination. Nuclear-powered Europa submarines will get us swimming with the singing Europan space whales in no time.
Europa is the best place to search the Solar System for life, and I’m excited to see what the upcoming Europa Orbiter mission turns up. And I’m even more excited about the possibility of any future lander missions.
It was a lot of fun wrapping my brain around a topic chosen by the fans. What topic would you like us to cover next? I’ve got a whole pocket of meteorites here. Put it in the comments below.
First, I want to thank everyone. It’s been a crazy race getting up to 200 episodes, but it’s been a blast all the way through. Thanks again for all your support and here’s to 200 more!
Weekly Space Hangout – June 26, 2015: Paul Sutter, CCAPP Visiting Fellow
Host: Fraser Cain (@fcain)
Special Guest: This week we welcome Paul Sutter, the CCAPP Visiting Fellow who works on the cosmic microwave background and large-scale structure.
Guests:
Jolene Creighton (@jolene723 / fromquarkstoquasars.com)
Brian Koberlein (@briankoberlein / briankoberlein.com)
Morgan Rehnberg (cosmicchatter.org / @MorganRehnberg )
Alessondra Springmann (@sondy)
Continue reading “Weekly Space Hangout – June 26, 2015: Paul Sutter, CCAPP Visiting Fellow”