For over sixty years, astronomers have been exploring the Universe for x-ray sources. Known to be associated with stars, clouds of super heated gas, interstellar mediums, and destructive events, the detection of cosmic x-rays is challenging work. In recent decades, astronomers have been benefited immensely from by the deployment of orbital telescopes like the Chandra X-ray Observatory.
Since it was launched on July 23rd, 1999, Chandra has been NASA’s flagship mission for X-ray astronomy. And this past week (on Thurs. March 30th, 2017), the Observatory accomplished something very impressive. Using its suite of advanced instruments, the observatory captured a mysterious flash coming from deep space. Not only was this the deepest X-ray source ever observed, it also revealed what could be an entirely new phenomenon.
Located in the region of the sky known as the Chandra Deep Field-South (CDF-S), this X-ray emission source appeared to have come from a small galaxy located approximately 10.7 billion light-years from Earth. It also had some remarkable properties, producing more energy in the space of a few minutes that all the stars in the galaxy combined.
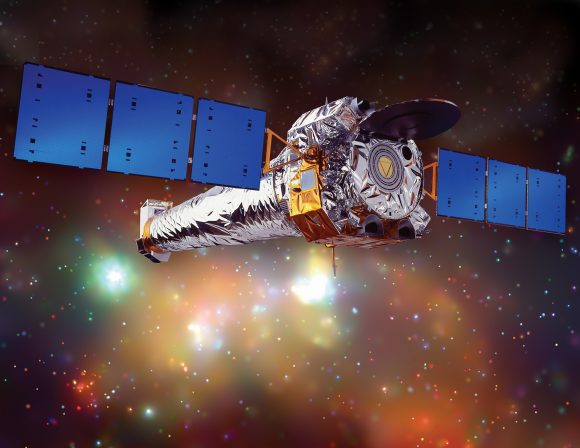
Originally detected in 2014 by a team of researchers from Penn State University and the Pontifical Catholic University of Chile in Santiago, Chile, this source was not even detected in the X-ray band at first. However, it quickly caught the team’s attention as it erupted and became 1000 brighter in the space of a few hours. At this point, the researchers began gathering data using Chandra’s Advanced CCD Imaging Spectronomer.
A day after the flare-up, the X-ray source had faded to the point that Chandra was no longer able to detect it. As Niel Brandt – the Verne M. Willaman Professor of Astronomy and Astrophysics at Penn State and part of the team that first observed it – described the discovery in a Penn State press release:
“This flaring source was a wonderful surprise bonus that we accidentally discovered in our efforts to explore the poorly understood realm of the ultra-faint X-ray universe. We definitely ‘lucked out’ with this find and now have an exciting new transient phenomenon to explore in future years.”
Thousands of hours of legacy data from the Hubble and Spitzer Space Telescopes was then consulted in order to determine the location of the CDF-S X-ray source. And though scientists were able to determine that the image of the X-ray source placed it beyond any that had been observed before, they are not entirely clear as to what could have caused it.
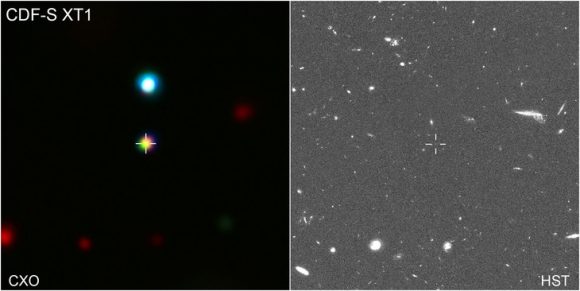
On the one hand, it could be the result of some sort of destructive event, or something scientists have never before seen. The reason for this has to do with the fact that X-ray bursts also come with a gamma-ray burst (GRB), which appears to be missing here. Essentially, GRBs are jetted explosions that are triggered by the collapse of a massive star or by the merger of two neutron stars (or a neutron star with a black hole).
Because of this, three possible explanations have been suggested. In the first, the CDF-S X-ray source is indeed the result of a collapsing star or merger, but the resulting jets are not pointed towards Earth. In the second, the same scenario is responsible for the x-ray source, but the GRB lies beyond the small galaxy. The third possible explanation is that the event was caused by a medium-sized black hole shredding a white dwarf star.
Unfortunately, none of these explanations seem to fit the data. However, these research team also noted that these possibilities are not that well understood, since none have been witnessed in the Universe. As Franz Bauer – an astronomer from the Pontifical Catholic University of Chile – said: “Ever since discovering this source, we’ve been struggling to understand its origin. It’s like we have a jigsaw puzzle but we don’t have all of the pieces.”
Not only has Chandra not observed any other X-ray sources like this one during the 17 years it has surveyed the CDF-S region, but no similar events have been observed by the space telescope anywhere in the Universe during its nearly two decades of operation. On top of that, this event was brighter, more short-lived, and occurred in a smaller, younger host galaxy than other unexplained X-ray sources.
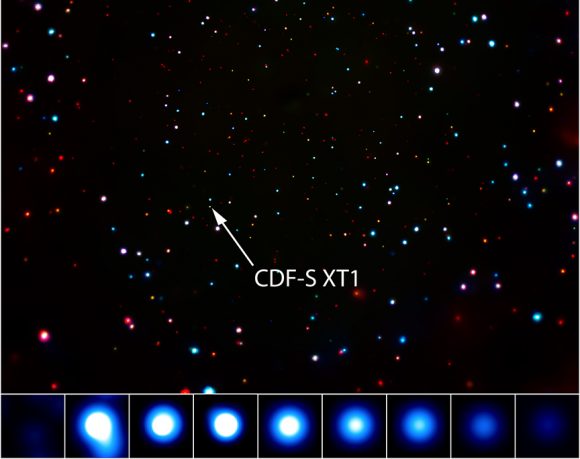
From all of this, the only takeaway appears to be that the event was likely the result of a cataclysmic event, like a neutron star or a white dwarf being torn apart. But the fact that none of the more plausible explanations seem to account for it’s peculiar characteristics would seem to suggest that astronomers may have witnessed an entirely new kind of cataclysmic event.
The team’s study – “A New, Faint Population of X-ray Transient“- is available online and will be published in the June 2017 issue of the Monthly Notices of the Royal Astronomical Society. In the meantime, astronomers will be sifting through the data acquired by Chandra and other X-ray observatories – like the ESA’s XMM-Newton and NASA’s Swift Gamma-Ray Burst Mission – to see if they can find any other instances of this kind of event.
And of course, future surveys conducted using Chandra and next-generation X-ray telescopes will also be on the lookout for these kind of short-lived, high-energy X-ray bursts. It’s always good when the Universe throws us a curve ball. Not only does it show us that we have more to learn, but it also teaches us that we must never grow complacent in our theories.
Be sure to check out this animation of the CDF-S X-ray source too, courtesy of the Chandra X-ray Observatory: