Greetings, fellow SkyWatchers! Are you ready for an mmm mmm good weekend? Well, the “m” stands for Messier and we’re off to study three of the late year’s finest… and a Herschel object as well! Don’t feel like taking out the telescope? Then don’t worry, because all of our weekend studies are easily done with even small binoculars! When ever you’re ready, I’ll see you in the dark…
Friday, December 4, 2009 – On this date in 1978, the Pioneer Venus Orbiter became the first spacecraft to orbit Venus. And, in 1996, the Mars Pathfinder mission was launched.
Tonight we’ll launch toward a bright open cluster known by many names: Herschel VII.32, Melotte 12, Collinder 23, and NGC 752. You’ll find it three finger-widths south (RA 01 57 41 Dec +37 47 06) of Gamma Andromedae.
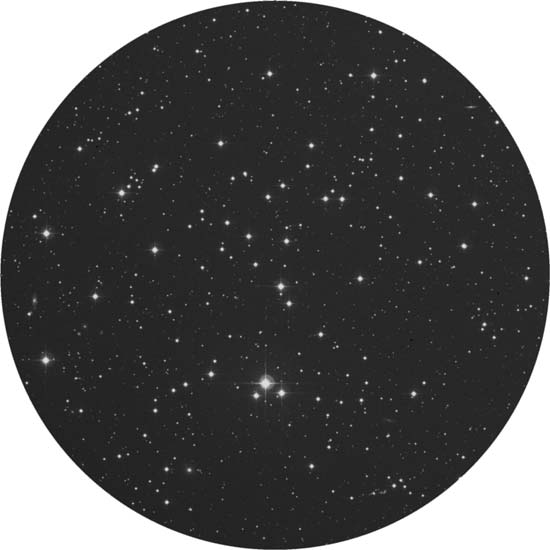
Under dark skies, this 5.7-magnitude open cluster can just be spotted with the unaided eye, is revealed in the smallest of binoculars, and can be completely resolved with a telescope. Chances are NGC 752 was discovered by Hodierna over 350 years ago, but it was not cataloged until Sir William gave it a designation in 1786. But give credit where credit is due, for it was Caroline Herschel who observed it on September 28, 1783! Containing literally scores of stars, galactic cluster NGC752 could be well over a billion years old and is strung out in chains and knots in an X pattern over a rich field. Take a close look at the southern edge for orange star 56; although this is a true binary star, the companion you see is merely optical. Enjoy this unsung symphony of stars tonight!
Saturday, December 5, 2009 – No one is certain, but it is believed that Werner Heisenberg was born on this date in 1901! Heisenberg was a physicist and philosopher who discovered a way to formulate quantum mechanics in terms of matrices. His uncertainty principle won him the Nobel Prize for Physics in 1932.
Is it gone yet? Nope. The Moon will rise a little later this evening, but we’re going to run ahead of it tonight and enjoy some studies in Auriga! Looking roughly like a pentagon in shape, Capella is the brightest of these stars. Due south of Capella is the second brightest star, El Nath. By aiming binoculars at El Nath, go north about one-third the distance between the two and enjoy all the stars! You will note two very conspicuous clusters of stars in this area, and so did Le Gentil in 1749.
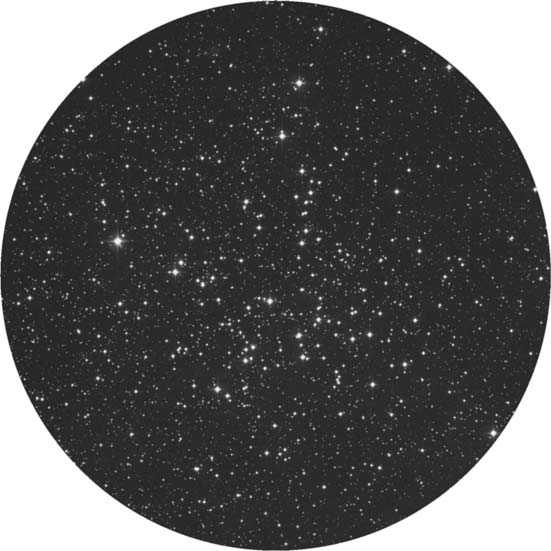
Binoculars will reveal the pair in the same field, as will telescopes using lowest power. The dimmest of these is M38 (RA 05 28 43 Dec +35 51 18), and it will appear vaguely cruciform in shape. At roughly 4,200 light-years away, the 100 or so fainter members will require larger aperture to resolve.
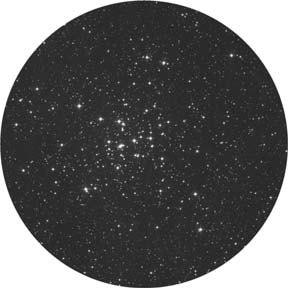
About 2.5 degrees to the southeast (RA 05 36 12 Dec +34 08 24) you will see the much brighter M36. More easily resolved in binoculars and small scopes, this ‘‘jewel box’’ galactic cluster is quite young and about 100 light-years closer!
Sunday, December 6, 2009 – Today we note the 1848 birth on this date of Johann Palisa. He discovered 122 asteroids with a 600 refractor, and all were visual observations. Check out some asteroids for yourself over the next few days as they approach easily noted objects. You’ll still find the asteroid Psyche close to Jupiter!
The Moon will be along shortly, but we still have time to set our sights about halfway between Theta Aurigae and El Nath. Our study object will be the open cluster M37 (RA 05 52 19 Dec+32 33 12).
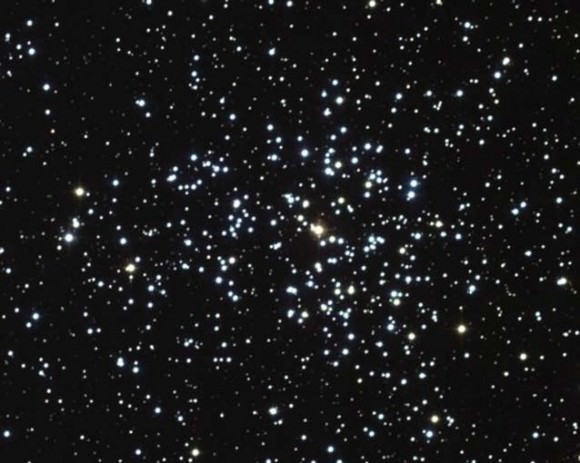
Apparently discovered by Messier himself in 1764, this galactic cluster will appear almost nebula-like to binoculars and very small telescopes, but comes to perfect resolution with larger instruments. About 4,700 light-years away and spanning a massive 25 light-years, M37 is often billed as the finest of the three Aurigan open clusters for bigger scopes. Offering beautiful resolvability, this one contains around 150 members down to magnitude 12 and has a total population in excess of 500.
What makes it unique? As you view, you will note the presence of several red giants. For the most part, open clusters are composed of stars that are all about the same age (usually young), but the brightest star in M37 appears orange in color and not blue! So what exactly is going on here? Apparently, some of these big, bright stars have evolved much faster, consuming their fuel at an incredible rate. Other stars in this cluster are still quite young on a cosmic scale, yet they all left the ‘‘nursery’’ at the same time! In theory, this allows us to judge the relative age of open clusters. For example, M36 is around 30 million years old and M38 about 40, but the presence of the red giants in M37 puts its estimated age at 150 million years!
Enjoy the weekend and keep a look out for stray members of the Geminid meteor shower!
This week’s awesome images are (in order of appearance): NGC 752, M38 and M36 (credit—Palomar Observatory, courtesy of Caltech), Johann Palisa (historical image) and M37 (credit—NOAO/AURA/NSF). We thank you so much!