[/caption]
For years, astronomer Karl Gebhardt and graduate student Jeremy Murphy at The University of Texas at Austin have been hunting for black holes — the dense concentration of matter at the centre of galaxies. Earlier this year, they made a record-breaking discovery. They found a black hole weighing 6.7 billion times the mass of our Sun in the centre of the galaxy M87.
But now they shattered their own record. Combining new data from multiple observations, they’ve found not one but two supermassive black holes that each weigh as much as 10 billion Suns.
“They just keep getting bigger,” Gebhardt said.
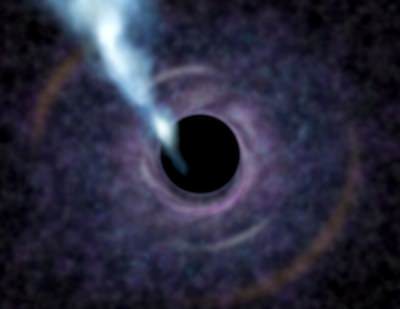
Black holes are made of extremely densely packed matter. They produce such a strong gravitational field that even light cannot escape. Because they can’t be seen directly, astronomers find black holes by plotting the orbits of stars around these giant invisible masses. The shape and size of these stars’ orbits can determine the mass of the black hole.
Exploding stars called supernovae often leave behind black holes, but these only weigh as much as the single star. Black holes billions of times the mass of our Sun have grown to be so big. Most likely, an ordinary black hole consumed another, captured huge numbers of stars and the massive amount of gas that they contain, or be the result of two galaxies colliding. The larger the collision, the more massive the black hole.
The supermassive black holes Gebhardt and Murphy have found are at the centres of two galaxies more than 300 million light years from Earth. One weighing 9.7 billion solar masses is located in the elliptical galaxy NGC 3842, the brightest galaxy in the Leo cluster of galaxies 320 million light years away in the direction of the constellation Leo. The other is as large or larger and sits in the elliptical galaxy NGC 4889, the brightest galaxy in the Coma cluster about 336 million light years from Earth in the direction of the constellation Coma Berenices.
Each of these black holes has an event horizon — the point of no return where nothing, not even light can escape their gravity — 200 times larger than the orbit of Earth (or five times the orbit of Pluto). That’s a mind-boggling 29,929,600,000 kilometres or 18,597,391,235 miles. Beyond the event horizon, each has a gravitational influence that extends over 4,000 light years in every direction.

For comparison, the black hole at the centre of our Milky Way Galaxy has an event horizon only one-fifth the orbit of Mercury — about 11,600,000 kilometres or 7,207,905 miles. These supermassive black holes are 2,500 times more massive than our own.
Gebhardt and Murphy found the supermassive black holes by combining data from multiple sources. Observations from the Gemini and Keck telescopes revealed the smallest, innermost parts of these galaxies while data from the George and Cynthia Mitchell Spectrograph on the 2.7-meter Harlan J. Smith Telescope revealed their largest, outmost regions.
Putting everything together to deduce the black holes’ mass was a challenge. “We needed computer simulations that can accommodate such huge changes in scale,” Gebhardt said. “This can only be done on a supercomputer.”
But the payoff doesn’t end with finding these massive galactic centre. The discovery has much more important implications. It “tells us something fundamental about how galaxies form” Gebhardt said.
These black holes could be the dark remnants of previously bright galaxies called quasars. The early universe was full of quasars, some thought to have been powered by black holes 10 billion Solar masses or more. Astronomers have been wondering where these supermassive galactic centres have since disappeared to.
Gebhardt and Murphy might have found a key piece in solving the mystery. Their two supermassive black holes might shed light on how black holes and their galaxies have interacted since the early universe. They may be a missing link between ancient quasars and modern supermassive black holes.
Source: McDonald Observatory Press Release.
Some guy also found The Black Hole. 😉
Hey, that idea is stolen from the movie Yellow Submarine featuring the Beatles. 😉
No, they stole that idea from Wile E. Coyote and Road Runner. 😉
Ok, we’re going to have to come up with a new name for these things. Extra-supermassive blackholes? Uber-supermassive? Mega-supermassive? Ultra-supermassive?
Hiper-supermassive is the correct term.
The obvious joke now that “hyper-supermassive” is the correct correct term.
Or perhaps the correct^2 term in a Taylor series approximation of correctness, around science blogs.
Apparently a black hole is “super-massive” if it has a density less than that of water. How would these black holes’ densities compare?
This hits the roof of my english. Do you mean to say you prefer “massed” over “massive”?
No no, what I mean is that the definition of “super-massive” (as opposed to a ‘normal’ black hole) depends on its average density (its volume being that within the Schwarzschild radius).
Apparently, the density of water is the arbitrary density which decides whether a black hole is super-massive or not. This is according to wikipedia:
http://en.wikipedia.org/wiki/Super_massive_black_hole
So, if higher mass black holes have lower average density, then we could set the density of, say, air (?) to classify black holes as “hyper-massive”
A preprint of the Nature paper has been posted on arXiv: http://arxiv.org/PS_cache/arxiv/pdf/1112/1112.1078v1.pdf
Table 4 lists over 60 galaxies where the mass of the SMBH has been determined. It seems only three galaxies listed here possess SMBHs smaller than the 4.1 million solar-mass BH in the Milky Way(M 32, M 64 & Circinus).
Our galaxy has a rather small SMBH compared to some galaxies. It is probably a good thing, for life could be pretty disruptive in a galaxy with a giga-solar mass BH.
LC
Depends on where the planet is located, of course. Earth is also placed quite far away from the centre.
On the other hand, there seems to be a correlation between the mass of a SMBH and AGN activity. A very active black hole might be lethal for the entire galaxy.
Does the amount of time that slows down increase with the mass of the black hole? If I could get from the Earth to a 10bn solar mass black hole in one day, then orbited it for one day (as per the watch on my wrist), then returned to Earth on the third day, how much time would have passed for someone who stayed on the Earth for three days versus the three days that elapsed for me?
Yes, the mass of the black hole, or any extremely large object for that matter, impacts the amount of time. From the pyramids of giza to the supermassive black hole at the center of our galaxy. I can’t tell you the exact numbers you’re looking for, but you should be able to calculate it using the numbers estimated for the time known for our supermassive black hole.
I feel more comfortable to use ratio here. (Maybe because english is not my native language.) The ratio of time dilation depends on velocity and acceleration, and according to general relativity that acceleration can be gravitational as well as inertial.
Gravitational acceleration depends on spacetime curvature. As a first approximation, the event horizon should happen at the same curvature (gravity) for all black holes (BHs), unless I am mistaken.
[For secondary effects, ask a general relativist. =D Among other things rotation and general relativity effects from that will change the naive picture.]
At a guess, it doesn’t depend on how massive the BH is assuming you orbit at the same curvature (gravity) conditions.
I got my facts confused, I shouldn’t have answered without knowing my stuff, apologies. Just started taking fascination into time acceleration. But I’m also interested in knowing, if anyone has the time to correct me 🙂
Hmm.. I think time dilation only depends on relative velocity, although you can calculate proper time with an acceleration using the Lorentz equations.
Agreed. And thanks, I see I didn’t express myself at all clearly!
for example, time goes by a billionth of a second different for people near the pyramids in comparison to people who are not. An extremely small difference. But if you orbited the supermassive black hole in the center of our universe for 5 years, and returned to Earth, 10 years would have passes here on Earth. Time drags on objects as a 4th dimension. The larger the object, the more drag.
There is no center of the universe.
LC
I think he had meant to say “the center of our [galaxy]”.
Yeah, sorry about that
Yeah, sorry about that
Maybe this discovery, that maybe most of the SMBH’s are mors massive than previously thought, will make dark matter less abundant in calculations?
No, we have enough observation of dark matter effects such as gravity lensing to see that it is (mostly) particulate.