There are many mysteries in the world of astronomy and a fair number relate to the processes during the end of the life of a super massive star. Throw in the complexity of collisions and you have a real head scratching problem on your hands. In 2017 colliding neutron stars were detected and the data has allowed a new simulation to be tested with predictions beautifully matching observation.
Neutron stars are stellar corpses no more than 10km or 20km across. They are thought to form when a supermassive star goes supernova at the end of its life and undergoes gravitational collapse. The collapse causes the remains to be compressed down to incredibly high densities, of the region 450 million billion kilograms per cubic metre (that’s equivalent to the density of an atomic nucleus). To put this into context, under the gravitational collapse, all the space between the components of atomic nuclei is squeezed out creating a gigantic neutron several kilometres across!
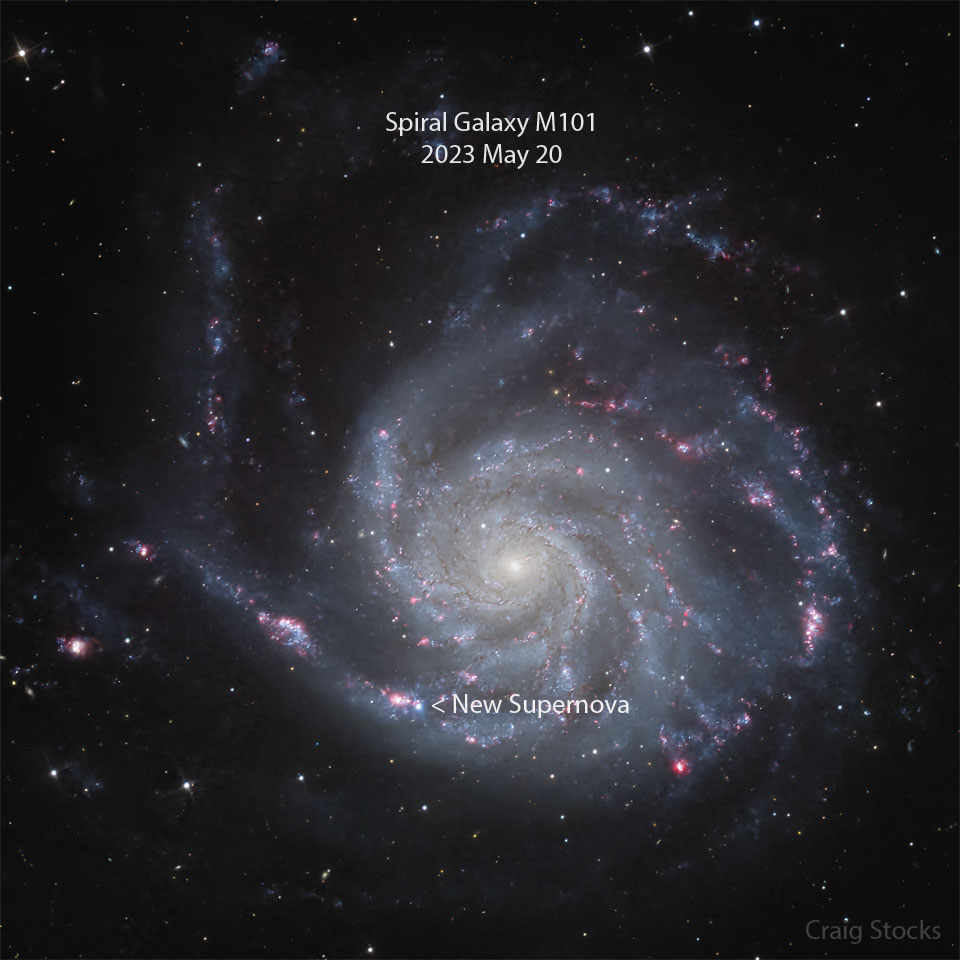
It seems quite a common occurence for neutron stars to orbit in binary systems and as they do, slowly eek away energy in the form of gravity waves. These waves are like those on the ocean instead propagate through the fabric of space-time. Eventually, sufficient energy is lost that the neutron stars collide and it is this that has allowed teams of astronomers to study the processes during some of the most extreme conditions found in the Universe.
An international team that involved the Max Planck Institute for Gravitational Physics and the University of Potsdam have used a new software tool to simulate the physical processes from neutron star mergers (otherwise known as a kilonova). The team also utilised X-ray observations, radio signals, nuclear physics calculations and even data from Earth based accelerators and for the first time plugged the whole lot into the simulations.
On 17 August the LIGO/Virgo team detected two neutron stars colliding in an elliptical galaxy in Hydra. The collision was identiifed from gravitational wave and gamma ray observations and by studying such high energy collisions we can learn more about the formation of heavy elements at extreme pressures and densities far greater than found in atomic nuclei.

The results were very promising with the predictions from the model matching observation. Now the team are running further observations with gravitational wave detectors as they hunt down the next neutron star merger to use the tool again and further enhance its model.
“To put this into context, under the gravitational collapse, all the space between the components of atomic nuclei is squeezed out creating a gigantic neutron several kilometres across!”
There is no such thing of course and notably the press has no such claim, I’m amazed to see this at Universe Today!
On the one hand, neutrons are composite elementary particle defined by having three valence quarks in a confined sea of quarks and gluons.
On the other, neutron star equation of state models exhibit gas – or at these pressures liquid – “polytropic” energy transfer thermodynamics. “Neutron stars are well modeled by polytropes with index between n = 0.5 and n = 1.” [“Polytrope”, Wikipedia]. Universe Today had a recent article on an exciting* find that (so far limited) statistics of neutron star densities prefer models where neutron liquids phase changes to quark liquid cores in the most massive neutron stars. [“The Most Massive Neutron Stars Probably Have Cores of Quark Matter”, JANUARY 3, 2024 BY BRIAN KOBERLEIN.] But even then there was no attempt to claim the quark liquid was a giant “neutron”, whatever that is supposed to mean.
*Exciting just not because of “quark stars” analogies, but because such a demonstration of a smooth, second order, QCD phase change in nature helps understand why the universe has not formed ‘QCD bubbles’ with different types of nucleons such as neutrons.