It seems unlikely that an ocean could persist on a world that never gets closer than 30 astronomical units from the Sun. But that’s the case with Pluto. Evidence shows that it has a sub-surface ocean between 100 to 180 km thick, at the boundary between the core and the mantle. Other Kuiper Belt Objects may be similar.
But time might be running out for these buried oceans, which will one day turn to ice.
For a long time, thinking about Pluto’s ocean was centered around one question: How did Pluto’s ocean survive to the present day? Embedded in that question is the accepted scenario for Pluto’s formation: the ice dwarf originated as a frozen ball of ice and rock. Some of that rock underwent radioactive decay, releasing enough heat to melt some of the ice, creating a sub-surface ocean covered with a layer of ice.
But a new study is urging astronomers to rethink Pluto’s origins.
The new study is titled “Evidence for a hot start and early ocean formation on Pluto.” The lead author is Carver Bierson, a graduate student at University of California, Santa Cruz. The paper is published in the journal Nature Geoscience.
This ‘hot start’ scenario says that Pluto didn’t start out as a frozen ball and then develop its ocean. Instead, the accretion of material during Pluto’s formation created enough heat to create the ocean as the dwarf planet itself was created. And despite Pluto’s great distance from the Sun, that ocean has persisted for billions of years.
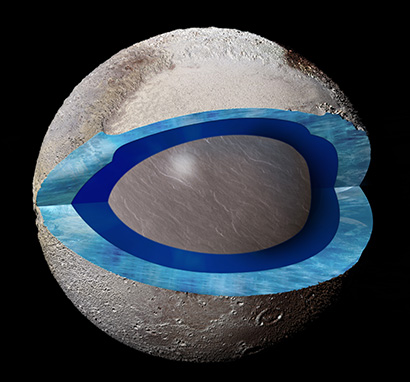
In this hot start scenario, radioactive decay came later. That decay didn’t create the sub-surface ocean; it just maintained it to this day, preventing all that water from freezing. Evidence from NASA’s New Horizons mission supports this scenario.
“For a long time people have thought about the thermal evolution of Pluto and the ability of an ocean to survive to the present day,” said coauthor Francis Nimmo, professor of Earth and Planetary Sciences at UC Santa Cruz. “Now that we have images of Pluto’s surface from NASA’s New Horizons mission, we can compare what we see with the predictions of different thermal evolution models, Nimmo said in a press release.
Unlike other liquids, water expands when it freezes, and contracts when it melts. This leaves telltale signs on the surface of Pluto. Those signs are different for a cold-start scenario vs. a hot-start scenario.
“If it started cold and the ice melted internally, Pluto would have contracted and we should see compression features on its surface, whereas if it started hot it should have expanded as the ocean froze and we should see extension features on the surface,” said lead author Bierson. “We see lots of evidence of expansion, but we don’t see any evidence of compression, so the observations are more consistent with Pluto starting with a liquid ocean.”
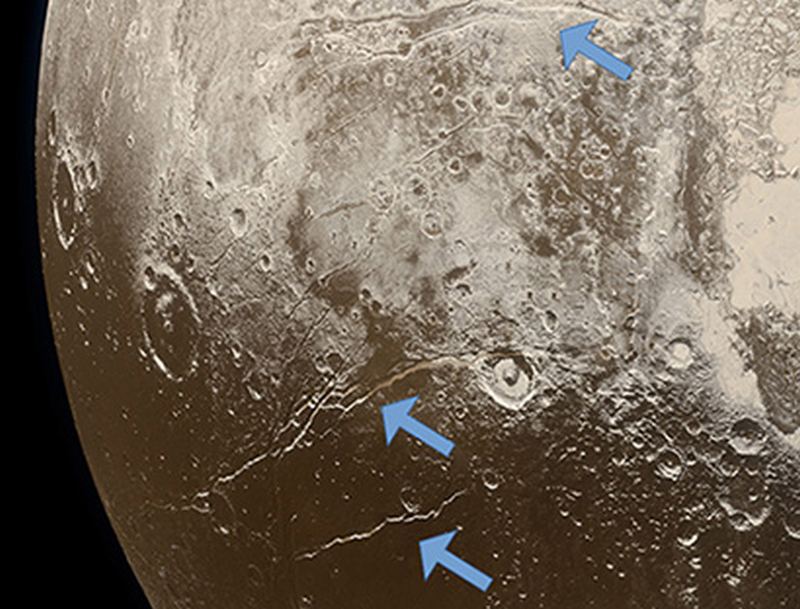
But the signs of the hot-start scenario are not simple to decipher. Pluto, like any body, has a complicated history that spans billions of years. It took some scientific sleuthing to figure this all out.
A cold-start Pluto would have a complicated evolution, leaving signs of thermal and tectonic evolution on its surface. If it started out cold, then was heated by radioactive decay, it would melt then eventually begin to refreeze again. This would leave telltale signs of expansion in later and modern times as ice melted and the water expanded. But it would leave evidence of contraction in ancient times.
The evidence would be different for a hot-start Pluto. In this scenario, evidence of extension would span the entire history of Pluto, since it had nowhere to go but cooler, meaning ongoing expansion. Of course, the further back in time scientists try to look, the more difficult it is to find evidence.
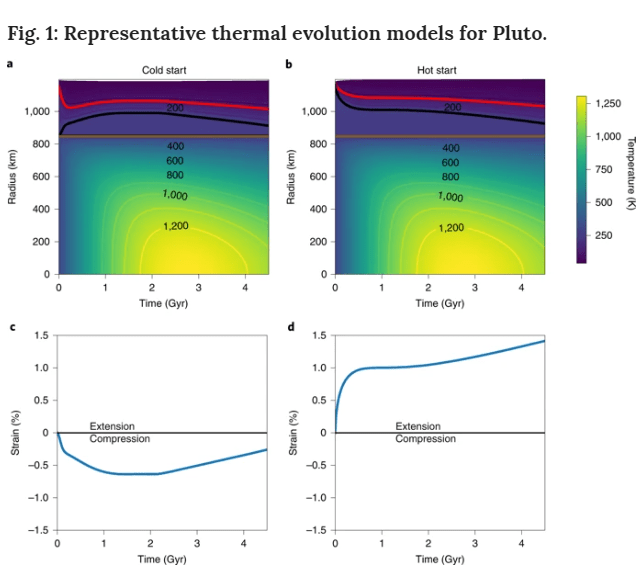
“The oldest surface features on Pluto are harder to figure out, but it looks like there was both ancient and modern extension of the surface,” Nimmo said.
But surface evidence is only one part of this study. The researchers also asked another pertinent question: was there enough energy to give Pluto its hot start?
“How Pluto was put together in the first place matters a lot for its thermal evolution.”
Francis Nimmo, Study Co-Author, Professor of Earth and Planetary Sciences at UC Santa Cruz
Pluto is much too distant from the Sun to get much heat from the star. It had only two sources of heat: heat from radioactive decay, and heat from new material bombarding the growing young planet.
When material strikes a body like young Pluto, the gravitational energy of the impact is converted to heat. Depending on the timeline of Pluto’s formation, Bierson’s calculations showed that impacts could have created enough heat to maintain the liquid water. But only if all of that heat was retained, which is unlikely.
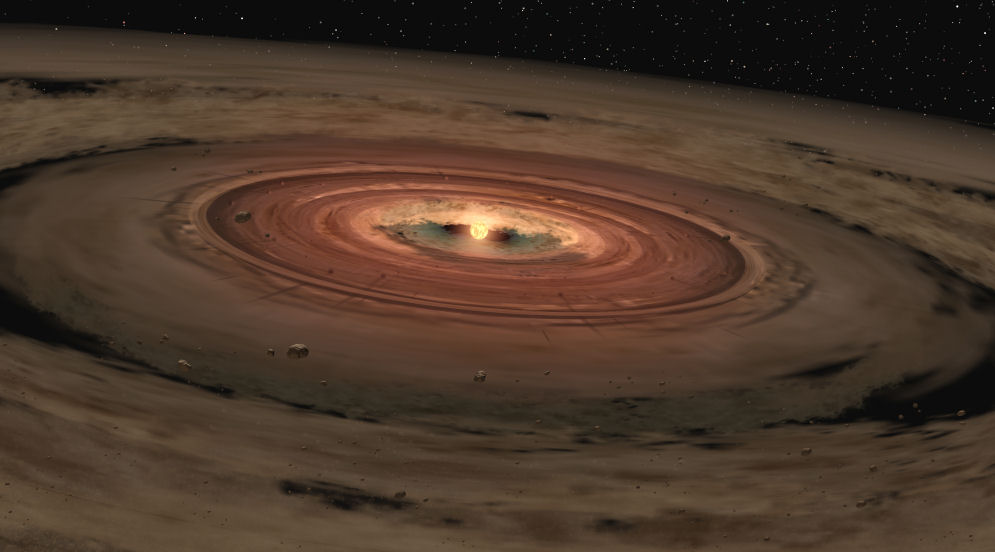
Some heat would inevitably escape to space. If Pluto grew slowly, accreting material on a longer time-scale, then much of the heat would’ve dissipated into space. But if new material was buried by other material more quickly, the heat would be trapped.
“How Pluto was put together in the first place matters a lot for its thermal evolution,” Nimmo said. “If it builds up too slowly, the hot material at the surface radiates energy into space, but if it builds up fast enough the heat gets trapped inside.”
There’s a critical time threshold for the hot-start scenario: 30,000 years. According to the researchers, if Pluto’s formation took 30,000 years or less, then the hot start is valid. There would not have been enough time for the initial heat to dissipate.
But if it took longer than that, and if Pluto accreted over millions of years, then the cold-start scenario fits the evidence. The only way the hot start scenario could have survived an accretion period that long is if there were impactors, that buried their heat deep beneath Pluto’s surface.
And that brings us to Sputnik Planitia. Sputnik Planitia (SP) is a large impact basin on the surface of Pluto, 1500 km (930 miles) in diameter. SP is composed of ice, primarily nitrogen ice. It’s likely a large impact basin that collected that ice over time after its formation.
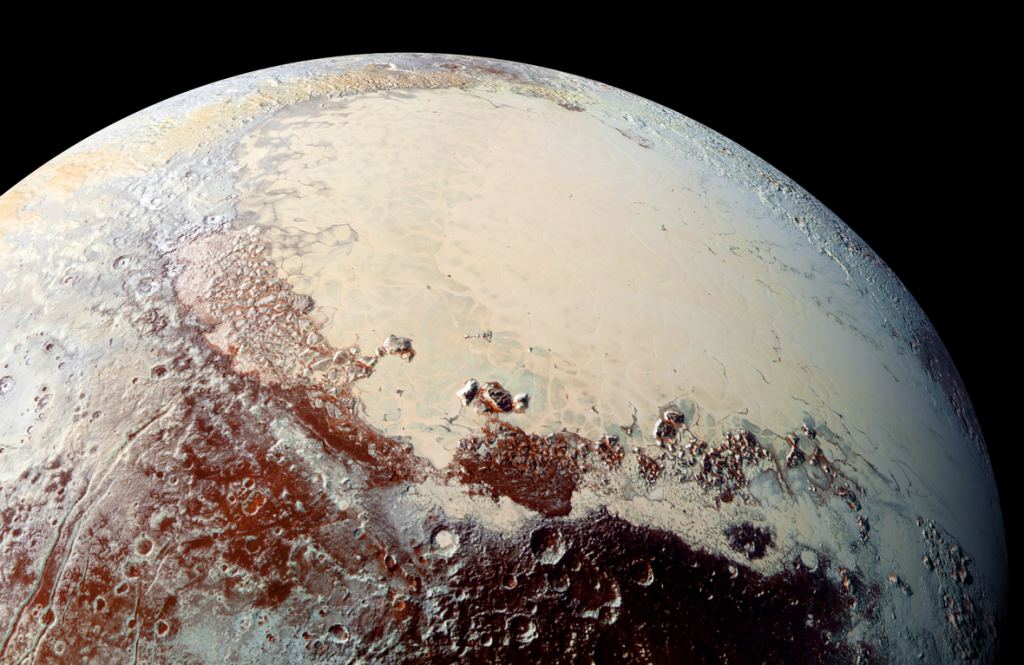
Sputnik Planitia provides some constraints for the hot-start and cold-start models. Scientists are uncertain of the exact depth of all the ice in SP, but a reasonable estimate is 10 km (6.2 miles), an enormous depth. For that depth to be accurate, there would have to be an elastic thickness in the lithosphere underneath SP of 40 km (25 miles). In a hot-start scenario, that required elastic thickness could be reached in about 100 million years after formation. In a cold-start scenario, the 40 km elastic thickness is never reached.
Pluto is a Kuiper Belt Object, so the results of this study might extend to other KBOs too. “Our results imply that initial liquid water oceans were a generic feature of larger dwarf planets in the Kuiper belt,” they wrote.
The authors also considered Charon’s potential to host a hot-start ocean. “…the hot start we envisage is probably relevant only for bodies of roughly half Pluto’s radius or more. Charon, at half Pluto’s radius, is therefore instructive. The absence of any compressional features on Charon suggests that Charon also underwent a hot start, though in this case the ocean is not expected to have survived to the present day.”

The bulk of KBOs are too small for oceans to persist this long. But for larger objects like Eris and Makamake, hot-start oceans might still exist. “Even in this cold environment so far from the sun, all these worlds might have formed fast and hot, with liquid oceans,” Bierson said.
But those oceans are doomed. If they have any potential to host life, and if there were anything living there, their forecast is not good. Eventually, radioactive decay will wind down, and the only source of heat will be gone. Any sub-surface oceans will inevitably freeze forever.
More:
- Press Release: Evidence supports ‘hot start’ scenario and early ocean formation on Pluto
- Research Paper: Evidence for a hot start and early ocean formation on Pluto
- Universe Today: NASA is Now Considering a Pluto Orbiter Mission