Since the “Golden Age of General Relativity” in the 1960s, scientists have held that much of the Universe consists of a mysterious invisible mass known as “Dark Matter“. Since then, scientists have attempted to resolve this mystery with a double-pronged approach. On the one hand, astrophysicists have attempted to find a candidate particle that could account for this mass.
On the other, astrophysicists have tried to find a theoretical basis that could explain Dark Matter’s behavior. So far, the debate has centered on the question of whether it is “hot” or “cold”, with cold enjoying an edge because of its relative simplicity. However, a new study conducted led by the Harvard-Smithsonian Center for Astrophysics (CfA)
This was based on cosmological simulations of galaxy formation using a model of a Universe that included interactive Dark Matter. The simulations were conducted by an international team of researchers from the CfA, MIT’s Kavli Institute for Astrophysics and Space Research, the Leibniz Institute for Astrophysics Potsdam, and multiple universities. The study recently appeared in the Monthly Notices of the Royal Astronomical Society.

When it comes right down to it, Dark Matter is appropriately named. For starters, it makes up about 84% of the mass of the Universe but neither emits, absorbs or reflects light or any other known form of radiation. Second of all, it has no electromagnetic charge and does not interact with other matter except through gravity, the weakest of the four fundamental forces.
Third, it not composed of atoms or their usual building blocks (i.e. electrons, protons, and neutrons), which contributes to its mysterious nature. As a result, scientists theorize that it must be made up of some new kind of matter that is consistent with the laws of the Universe but does not show up in conventional particle physics research.
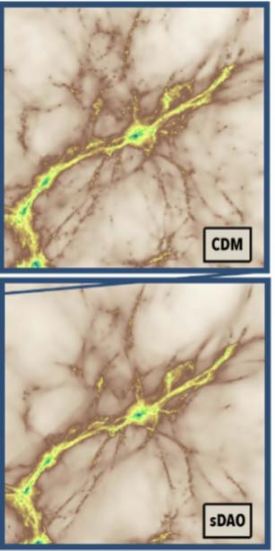
Regardless of its true nature, Dark Matter has had a profound influence on the evolution of the cosmos since about 1 billion years after the Big Bang onward. In fact, it is believed to have played a key role in everything from the formation of galaxies to the distribution of the Cosmic Microwave Background (CMB) radiation.
What’s more, cosmological models that take into account the role played by Dark Matter are backed up by observations of these two very different types of cosmic structures. Also, they are consistent with cosmic parameters like the rate at which the Universe is expanding, which is itself influenced by a mysterious, invisible force (known as “Dark Energy“).
Currently, the most widely accepted models of Dark Matter presume that it does not interact with any other kinds of matter or radiation (including itself) beyond the influence of gravity – i.e. that it is “cold”. This is what is known as the Cold Dark Matter (CDM) scenario, which is often combined with the theory of Dark Energy (represented by Lambda) in the form of the LCDM cosmological model.
This theoretical form of Dark Matter is also referred to as
“[CDM] is the most well-tested and the preferred model. This is primarily because over the last four decades or so, people have been working hard to make predictions using cold Dark Matter as the standard paradigm – these are then compared to real data – with the finding that, in general, this model is able to reproduce a wide range of observed phenomena across a wide range of scales.”
As he describes it, the cold Dark Matter scenario became the front-runner after numerical simulations of cosmic evolution were conducting using “hot Dark Matter” – in this case, the neutrino. These are subatomic particles that are very similar to an
These simulations showed that the predicted distributions looked nothing like the Universe does today,” Bose added. “For that reason, the opposite limit began to be considered, particles that have barely any velocity when they are born (aka. “cold”). Simulations that included this candidate fit modern observations of the Universe much more closely.
“After performing the same galaxy clustering tests as before, astronomers found a startling agreement between the simulated and observed universes. In the subsequent decades, the cold particle has been tested through more rigorous, non-trivial tests than simply galaxy clustering, and it has generally passed each of these with flying colors.”
Another source of appeal is the fact that cold Dark Matter (at least theoretically) ought to be detectable either directly or indirectly. However, this is where the CDM runs into trouble since all attempts at detecting a single particle so far have failed. As such, cosmologists have taken to consider other possible candidates that would have even smaller levels of interaction with other matter.
This is what Sownak Bose, an astronomer with the CfA, sought to determine with his team of researchers. For the sake of their study, they focused on a “warm” Dark Matter candidate. This type of particle would have the ability to subtly interact with very light particles that move close to the speed of light, though less so than the more interactive “hot” variety.
In particular, it could be capable of interacting with neutrinos, the former front-runner for the HDM scenario. Neutrinos are thought to have been very prevalent during the hot early Universe, so the presence of interacting Dark Matter would have had a strong influence.

“In this class of models, the Dark Matter particle is allowed to have a finite (but weak) interaction with a radiative species like photons or neutrinos,” said Dr. Bose. “This coupling leaves a rather unique imprint in the ‘lumpiness’ of the Universe at early times, which is quite a lot different to what might be expected if the Dark Matter was a cold particle.”
To test this, the team ran state-of-the-art cosmological simulations in the supercomputing facilities at Harvard and the University of Iceland. These simulations considered how galaxy formation would be affected by the presence of both warm and Dark Matter from about 1 billion after the Big Bang to 14 billion years (roughly the present). Said Dr. Bose indicated:
“[W]e ran computer simulations to generate realizations of what this Universe might look like after 14 billions years of evolution. In addition to modeling the Dark Matter component, we also included state-of-the-art prescriptions for star formation, the effects of supernovae and black holes, the formation of metals etc.”
The team then compared the results to each other to identify characteristic signatures that would distinguish one from the other. What they found was that for many of simulations the effects of this interactive Dark Matter were too small to be noticeable. However, they were present in some distinct ways, particularly in the way that distant galaxies are distributed throughout space.

This observation is especially interesting because it can be tested in the future using next-generation instruments. “The way to do this is to map the lumpiness of the Universe at these early times by looking at the distribution of hydrogen gas,” Dr. Bose explained. “Observationally, this is a well-established technique: we can probe neutral hydrogen in the early universe by looking at the spectra of distant galaxies (usually quasars).”
In short, light traveling to us from distant galaxies has to pass through the intergalactic medium. If there is a lot of neutral hydrogen in the intervening medium, the emission lines from the galaxy will be partially absorbed, whereas they will be unimpeded if there is little. If Dark Matter is truly cold, it will show up in the form of a much “lumpier” distribution of hydrogen gas, whereas a WDM scenario will result in oscillating lumps.
Currently, astronomical instruments do not have the necessary resolution to measure hydrogen gas oscillations in the early Universe. But as Dr. Bose indicated, this research could provide impetus for new experiments and new facilities that would be capable of making these observations.
For instance, IR instrument like the James Webb Space Telescope (JWST) could be used to create new maps of the distribution of hydrogen gas absorption. These maps would be able to either confirm the influence of interactive Dark Matter or rule it out as a candidate. It is also hoped that this research will inspire people to think of candidates beyond those that have already been considered.
In the end, Dr. Bose said, the real value comes from the fact that these kinds of theoretical predictions can spur observations into new frontiers and tests the limits of what we think we know. “And that’s all that science is really,” he added, “making a prediction, proposing a method for testing it, performing the experiment and then constraining/ruling out the theory!”
Definitely interesting. Many great discoveries have been made by sort of looking where no one looked before or following a counterintuitive path. So the idea of a warm dark matter that interacts uniquely with hydrogen is intriguing. I guess in time we’ll see if it has legs.