For many reasons, Venus is sometimes referred to as “Earth’s Twin” (or “Sister Planet”, depending on who you ask). Like Earth, it is terrestrial (i.e. rocky) in nature, composed of silicate minerals and metals that are differentiated between an iron-nickel core and silicate mantle and crust. But when it comes to their respective atmospheres and magnetic fields, our two planets could not be more different.
For some time, astronomers have struggled to answer why Earth has a magnetic field (which allows it to retain a thick atmosphere) and Venus do not. According to a new study conducted by an international team of scientists, it may have something to do with a massive impact that occurred in the past. Since Venus appears to have never suffered such an impact, its never developed the dynamo needed to generate a magnetic field.
The study, titled “Formation, stratification, and mixing of the cores of Earth and Venus“, recently appeared in the scientific journal Earth and Science Planetary Letters. The study was led by Seth A. Jacobson of Northwestern University, and included members from the Observatory de la Côte d’Azur, the University of Bayreuth, the Tokyo Institute of Technology, and the Carnegie Institution of Washington.

For the sake of their study, Jacobson and his colleagues began considering how terrestrial planets form in the first place. According to the most widely-accepted models of planet formation, terrestrial planets are not formed in a single stage, but from a series of accretion events characterized by collisions with planetesimals and planetary embryos – most of which have cores of their own.
Recent studies on high-pressure mineral physics and on orbital dynamics have also indicated that planetary cores develop a stratified structure as they accrete. The reason for this has to do with how a higher abundance of light elements are incorporated in with liquid metal during the process, which would then sink to form the core of the planet as temperatures and pressure increased.
Such a stratified core would be incapable of convection, which is believed to be what allows for Earth’s magnetic field. What’s more, such models are incompatible with seismological studies that indicate that Earth’s core consists mostly of iron and nickel, while approximately 10% of its weight is made up of light elements – such as silicon, oxygen, sulfur, and others. It’s outer core is similarly homogeneous, and composed of much the same elements.
As Dr. Jacobson explained to Universe Today via email:
“The terrestrial planets grew from a sequence of accretionary (impact) events, so the core also grew in a multi-stage fashion. Multi-stage core formation creates a layered stably stratified density structure in the core because light elements are increasingly incorporated in later core additions. Light elements like O, Si, and S increasingly partition into core forming liquids during core formation when pressures and temperatures are higher, so later core forming events incorporate more of these elements into the core because the Earth is bigger and pressures and temperatures are therefore higher.
“This establishes a stable stratification which prevents a long-lasting geodynamo and a planetary magnetic field. This is our hypothesis for Venus. In the case of Earth, we think the Moon-forming impact was violent enough to mechanically mix the core of the Earth and allow a long-lasting geodynamo to generate today’s planetary magnetic field.”
To add to this state of confusion, paleomagnetic studies have been conducted that indicate that Earth’s magnetic field has existed for at least 4.2 billion years (roughly 340 million years after it formed). As such, the question naturally arises as to what could account for the current state of convection and how it came about. For the sake of their study, Jacobson and his team considering the possibility that a massive impact could account for this. As Jacobson indicated:
“Energetic impacts mechanically mix the core and so can destroy stable stratification. Stable stratification prevents convection which inhibits a geodynamo. Removing the stratification allows the dynamo to operate.”
Basically, the energy of this impact would have shaken up the core, creating a single homogeneous region within which a long-lasting geodynamo could operate. Given the age of Earth’s magnetic field, this is consistent with the Theia impact theory, where a Mars-sized object is believed to have collided with Earth 4.51 billion years ago and led to the formation of the Earth-Moon system.
This impact could have caused Earth’s core to go from being stratified to homogeneous, and over the course of the next 300 million years, pressure and temperature conditions could have caused it to differentiate between a solid inner core and liquid outer core. Thanks to rotation in the outer core, the result was a dynamo effect that protected our atmosphere as it formed.
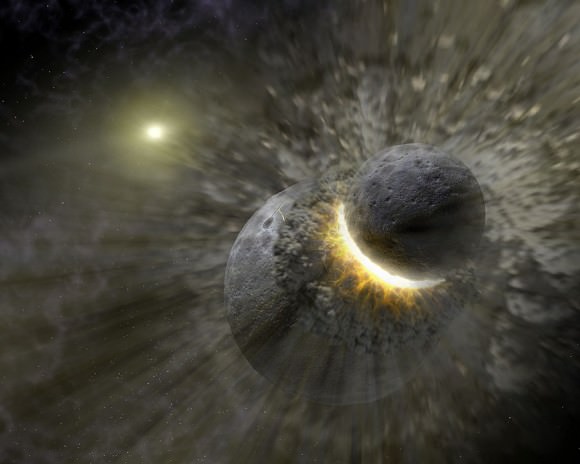
The seeds of this theory were presented last year at the 47th Lunar and Planetary Science Conference in The Woodlands, Texas. During a presentation titled “Dynamical Mixing of Planetary Cores by Giant Impacts“, Dr. Miki Nakajima of Caltech – one of the co-authors on this latest study – and David J. Stevenson of the Carnegie Institution of Washington. At the time, they indicated that the stratification of Earth’s core may have been reset by the same impact that formed the Moon.
It was Nakajima and Stevenson’s study that showed how the most violent impacts could stir the core of planets late in their accretion. Building on this, Jacobson and the other co-authors applied models of how Earth and Venus accreted from a disk of solids and gas about a proto-Sun. They also applied calculations of how Earth and Venus grew, based on the chemistry of the mantle and core of each planet through each accretion event.
The significance of this study, in terms of how it relates to the evolution of Earth and the emergence of life, cannot be understated. If Earth’s magnetosphere is the result of a late energetic impact, then such impacts could very well be the difference between our planet being habitable or being either too cold and arid (like Mars) or too hot and hellish (like Venus). As Jacobson concluded:
“Planetary magnetic fields shield planets and life on the planet from harmful cosmic radiation. If a late, violent and giant impact is necessary for a planetary magnetic field then such an impact may be necessary for life.”
Looking beyond our Solar System, this paper also has implications in the study of extra-solar planets. Here too, the difference between a planet being habitable or not may come down to high-energy impacts being a part of the system’s early history. In the future, when studying extra-solar planets and looking for signs of habitability, scientists may very well be forced to ask one simple question: “Was it hit hard enough?”
Further Reading: Earth Science and Planetary Letters
It’s amazing to realise that all life on Earth may owe its existence to an interplanetary collision which (1) melted the core to create a liquid metallic dynamo so that Earth would be protected from space radiation by an intense magnetic field, and (2) led to the creation of the Moon which stabilized the Earth and caused tides.
What are the odds against this happening? In other words, how rare is this in the universe? You could be forgiven for attributing this to an ‘intelligent designer’ – not that I do.
Maybe Venus doesn’t have the same amount of metals, therefore reducing the amount of magnetic energy, therefore shrinking the magnetosphere. So Venus might have a magnetosphere, but very small.
The universe is incomprehensibly vast, filled with an inconceivably large amount of matter. When considering such large, large numbers, the only logical conclusion is even the rarest of things, even earth-like development, must be boringly common.
We might not be able to observe that from our own tiny little corner of the sky, but we should at least concede that, given the sheer number of opportunities available for Earth’s history to be repeated over and over and over, it’s less reasonable to assume Earth and the circumstances that created us is unique. It makes much less sense to adopt the position that in all the wide universe, we are hopelessly alone.
Agreed – Nature is remarkably consistent. Simply because we evolved within this Earth-Moon system, it is sufficient to say that we are not alone, whether you define “alone” based on sentience, planetary habitability, or even being bilaterally-symmetric bipeds.
The do not seem to have given much attention to the spin of Venus which i very slow compared to Earth. This I am sure has a large effect on generation of a magnetic field.
Also I think it is time to revise the mechanism for planetary formation. The current view seems to be that particles in the ring of debris surrounding a newly formed star accreted into clumps through gravity and eventually these clumps attracted each other till sufficient gathered in one place to form a planet.
However we know that most if not all planes have a metal core, mainly iron and nickel. The current suggestion is that, at some point in the formation, all this iron and nickel somehow dropped into the core. This has always bothered me. How come only the iron and nickel dropped? What triggered it?
It seems to me more likely that the iron/nickel cores formed first and acted as gravitational attractors for the other materials. An accretion disc around a forming star has a lot of iron and nickel from the supernova which preceded the star formation and there is no reason why a good portion of it could not be in molecular form. It has to be in molecular form to be magnetic.
The motion of the disc through the magnetic field surrounding the forming star will create eddies and currents. This COULD magnetise the molecular iron and nickel and we know how rapidly magnetised particles will seek each other out and clump.
This seems to me a more efficient way to form cores on which other materials can accrete through gravity and solve the messy problem of how iron/nickel are required to ‘drop’ to the core after the formation of a planet.
The materials which accrete later will depend on where in the disc the metallic core lies, ergo the formation of rocky planets and gas planets but all forming around a metal core.
https://wordpress.com/view/rathkennamikeblog.wordpress.com
It’s an interesting notion…however, the reason that the iron and nickel “sank” to form the core is because they are more dense than silicate minerals, water, hydrocarbons, etc. Once the interior is hot enough from impacts and increasing gravitational pressure as it gets bigger, then there is enough atomic mobility for the more dense elements to differentiate from the others (the iron and nickel sinks while the silicates rise, being displaced by the more dense elements).
I agree, however, that the extremely low rotational period of Venus probably has a lot to do with it. A big clue is that it actually rotates slightly retrograde, which suggests a powerful, glancing impact –
perhaps in the same epoch in which Theia is hypothesized to have collided with Earth. As it is now, a “day” on Venus is longer than its “year”, and the Sun rises in the West and sets in the East.