Astrophysicists studying the expansion of the Universe with the largest galaxy catalogs ever assembled are ushering in an exciting era of precision cosmology. Last week, the Sloan Digital Sky Survey (SDSS) issued its final public data release, and scientists working in its largest program, the Baryon Oscillation Spectroscopic Survey (BOSS) also presented their final results at the American Astronomical Society meeting in Seattle, Washington.
By mapping over 10,000 square degrees — 25% of the sky — BOSS is “measuring our universe’s accelerated expansion with the world’s largest extragalactic redshift survey,” according to SDSS-III Director Daniel Eisenstein of the Harvard-Smithsonian Center for Astrophysics. The BOSS results include new and precise measurements of the universe’s expansion rate (called the “Hubble constant”) and matter density, which includes dark matter, stars, gas, and dust.
BOSS conducted its observations at 2.5-meter Sloan Foundation Telescope at Apache Point Observatory in New Mexico, producing spectra and spatial positions for 1.5 million galaxies and 300,000 quasars in a volume equivalent to a cube with length 8.5 billion light-years on a side (see image above). Astronomers used this rich dataset to map the objects’ distributions and to detect the characteristic scale imprinted by baryon acoustic oscillations in the early universe. Sound waves propagate outward with time, like ripples spreading in a pond, and are indicated by a large-scale clustering signal in the positions of galaxies relative to each other (see illustration below). By analyzing this signal at different times, it is possible to study the behavior of the mysterious “dark energy” causing the accelerating expansion of the universe.
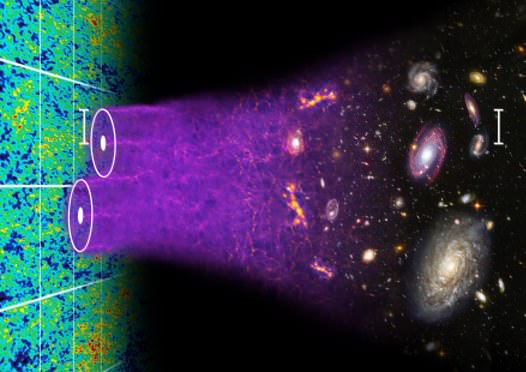
In BOSS’s final results, hundreds of scientists in the international collaboration measured this scale with unprecedented precision. In particular, Ashley Ross from Ohio State University presented results that demonstrated the power of combining an analysis of the transverse and line-of-sight distributions of galaxies. In a paper by Eric Aubourg and collaborators, BOSS astronomers measured the cosmic distance scale of galaxies in the “local” universe and of quasars in the distance universe with impressively small systematic errors—at less than the 1% level—when combined with cosmic microwave background constraints. Their cosmological analysis yields a measurement of the Hubble constant and of the matter density of the universe consistent with a “flat” cold dark matter cosmology with a cosmological constant (see below). Cosmological models including curvature, evolving dark energy, or massive neutrinos are not completely ruled out but are less supported by the data than before. Other results from the collaboration will be submitted for publication in the coming months.
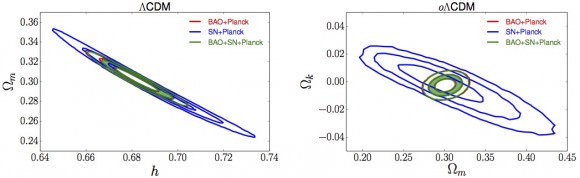
The BOSS dataset “represents the gold standard in mapping out the network of galaxies that comprises the large-scale structure of the Universe…The data enables us to trace, with greater precision than ever before, the presence of dark energy, the behaviour of gravity on cosmic scales, and the effect of massive neutrinos,” says Chris Blake of Swinburne University, not affiliated with the collaboration.
Where will the BOSS team go from here? The collaboration has begun work on SDSS-IV, whose six-year mission includes an ambitious extended BOSS (eBOSS) survey. According to eBOSS Targeting Coordinator Jeremy Tinker of New York University, eBOSS observations of over 700,000 quasars will precisely measure the distance scale “at a much higher redshift regime that is not covered by current large-scale surveys.”
You can read more about BOSS and updates about the three other componenets of the SDSS in our previous article here.
SDSS website
(Full disclosure: Ramin Skibba had been a member of the BOSS collaboration during 2010-2012.)