Decades after its momentous launch, the ever popular Hubble Space Telescope merrily continues its trajectory in low-earth orbit, and it still enables cutting-edge science. Astronomers utilized Hubble and its instruments over the years to obtain iconic images of the Crab Nebula, the Sombrero Galaxy, the Ultra Deep Field, and many others that captured the public imagination. Eventually its mission will end, and people need to plan for the next telescope and the next next telescope. But what kinds of space exploration do scientists want to engage in 20 years from now? What technologies will they need to make it happen?
A consortium of physicists and astronomers attempt to answer these questions as they put forward and promote their bold proposal for a giant high-resolution telescope for the next generation, which would observe numerous planets, stars, galaxies and the distant universe in stunning detail. In addition to encouraging support for scientific discoveries that could be made, the telescope’s advocates also must investigate the potential technical challenges involved in constructing and launching it. An event organized at a SPIE optics and photonics conference in San Diego, California on Tuesday served as another step in this long-term process.
The Association of Universities for Research in Astronomy (AURA), an influential organization of astronomers and physicists from 39 mostly US-based institutions, which operates telescopes and observatories for NASA and the National Science Foundation, laid out its proposal of a multi-wavelength High-Definition Space Telescope (HDST) in a new report last month. Julianne Dalcanton of the University of Washington and Sara Seager of the Massachusetts Institute of Technology—veteran astronomers with impressive knowledge and experience with galactic and planetary science—led the committee who researched and wrote the 172-page document.
“It’s the science community staking out a vision for what’s the next thing to do,” said Phil Stahl, former SPIE president and senior physicist at NASA’s Marshall Space Flight Center. Speaking at the optics and photonics conference about the telescope provided “an opportunity to speak to the people who will be building it,” as many of the audience work on instrumentation.
As the HDST’s name suggests, its 12-meter wide segmented mirror would give it much higher resolution than any current or upcoming telescopes, allowing astronomers to focus on many Earth-like “exoplanets” orbiting stars outside our solar system up to 100 light-years away, resolve stars even in the Andromeda Galaxy, and image faraway galaxies dating back 10 billion years of cosmic time into our universe’s past. The 24x increased sharpness compared to Hubble and the upcoming James Webb Space Telescope is similar to the dramatic improvement of an UltraHD TV over a standard television, according to Marc Postman, an astronomer at the Space Telescope Science Institute.
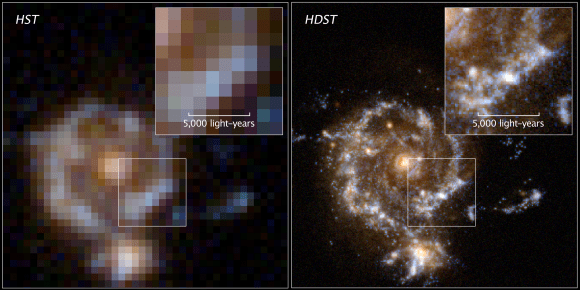
In particular, “exoplanets are the main science driver for the HDST,” said Seager. “Are there other planets like Earth, and are there signs of life on them?” Her and her colleagues’ excitement came through as she explained that, if the telescope comes to fruition, they predict it would find dozens, if not hundreds, of Earth-like planets in the habitable zone. They would look for evidence of oxygen and water vapor as well, transforming astronomers’ knowledge of such planets, currently limited to only 1 or 2 candidates detected by the Kepler telescope.
The Hubble telescope required 20 years of planning, technological development, and budget allocations before it was launched in 1990. Planning for NASA’s James Webb Space Telescope (JWST), which was also first proposed by AURA, began not long afterward. Rome wasn’t built in a day, but many years of preparations and research will come to fruition as it is set to launch in 2018. Its scientists and engineers hope that, like Hubble, it will produce spectacular images with its infrared cameras, become a household name, and expand our understanding of the universe.
Nevertheless, James Webb has been plagued by a ballooning budget and numerous delays, and Congress nearly terminated it in 2011. The telescope proved controversial even among some astronomers and space exploration advocates. As scientists develop the next generation of telescopes, JWST remains the multi-ton multi-billion-dollar elephant in the room. David Redding of Jet Propulsion Laboratory was quick to point out that, “for Hubble, almost every technology had to be invented!” For the proposed HDST, the task appears less daunting.
Nonetheless, scientists have technological challenges and difficult questions to look forward to. For example, they must choose among multiple competing designs and consider different methods for getting the telescope into space, possibly utilizing the Space Launch System (SLS). They also expect to leverage research on JWST’s sunshield, which will be necessary to keep the proposed telescope at an extremely stable temperature, and on its detectors, when developing optimized gigapixel-class cameras. Vibrational stability on the order of one trillionth of a meter will present an additional challenge for them.
If the astronomical community comes on board and prioritizes this project for the next decade, then it likely would be designed and constructed in the 2020s and then launched in the 2030s. In the meantime, they will need major investments of funding, research and development. According to Seager, it will certainly be worth it “to observe the whole universe at 100 parsec-scale resolution” and “discover dozens of Earths.” Adding emphasis, “that’s the killer app,” Postman concluded.