Thanks the Voyager missions and the more recent flybys conducted by the Cassini space probe, Saturn’s system of moons have become a major source of interest for scientists and astronomers. From water ice and interior oceans, to some interesting surface features caused by impact craters and geological forces, Saturn’s moons have proven to be a treasure trove of discoveries.
This is particularly true of Saturn’s moon Tethys, also known as a “Death Star Moon” (because of the massive crater that marks its surface). In addition to closely resembling the space station out of Star Wars lore, it boasts the largest valleys in the Solar System and is composed mainly of water ice. In addition, it has much in common with two of its Cronian peers, Mimas and Rhea, which also resemble a certain moon-size space station.
Discovery and Naming:
Originally discovered by Giovanni Cassini in 1684, Tethys is one of four moons discovered by the great Italian mathematician, astronomer, astrologer and engineer between the years of 1671 and 1684. These include Rhea and Iapetus, which he discovered in 1671-72; and Dione, which he discovered alongside Tethys.
Cassini observed all of these moons using a large aerial telescope he set up on the grounds of the Paris Observatory. At the time of their discovery, he named the four new moons “Sider Lodoicea” (“the stars of Louis”) in honor of his patron, king Louis XIV of France.
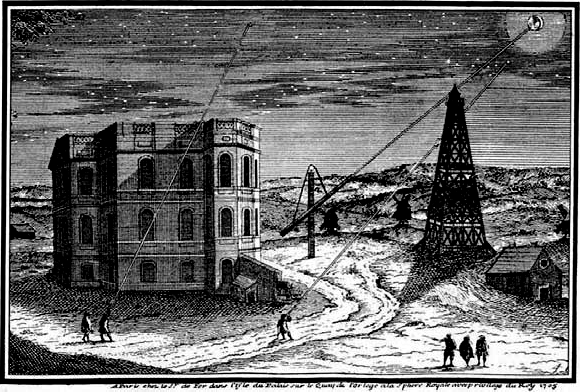
The modern names of all seven satellites of Saturn come from John Herschel (son of William Herschel, discoverer of Mimas and Enceladus). In his 1847 treatise Results of Astronomical Observations made at the Cape of Good Hope, he suggested that all should be named after the Titans – the brothers and sisters of Cronos – from Greek mythology.
Size, Mass and Orbit:
With a mean radius of 531.1 ± 0.6 km and a mass of 6.1745 ×1020 kg, Tethys is equivalent in size to 0.083 Earths and 0.000103 times as massive. Its size and mass also mean that it is the 16th-largest moon in the Solar System, and more massive than all known moons smaller than itself combined. At an average distance (semi-major axis) of 294,619 km, Tethys is the third furthest large moon from Saturn and the 13th most distant moon over all.
Tethys’ has virtually no orbital eccentricity, but it does have an orbital inclination of about 1°. This means that the moon is locked in an inclination resonance with Saturn’s moon Mimas, though this does not cause any noticeable orbital eccentricity or tidal heating. Tethys has two co-orbital moons, Telesto and Calypso, which orbit near Tethys’s Lagrange Points.
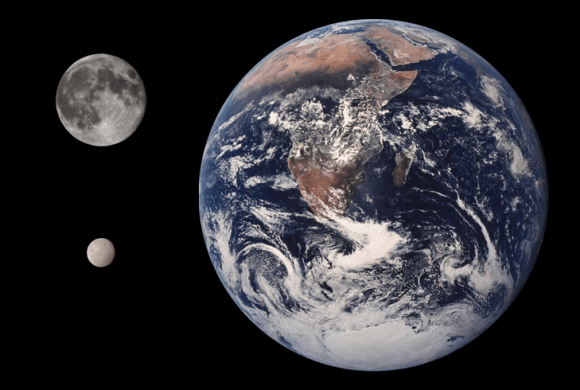
Tethys’ orbit lies deep inside the magnetosphere of Saturn, which means that the plasma co-rotating with the planet strikes the trailing hemisphere of the moon. Tethys is also subject to constant bombardment by the energetic particles (electrons and ions) present in the magnetosphere.
Composition and Surface Features:
Tethys has a mean density of 0.984 ± 0.003 grams per cubic centimeter. Since water is 1 g/cm3, this means that Tethys is comprised almost entirely of water ice. In essence, if the moon were brought closer to the Sun, the vast majority of the moon would sublimate and evaporate away.
It is not currently known whether Tethys is differentiated into a rocky core and ice mantle. However, given the fact that rock accounts for less 6% of its mass, a differentiated Tethys would have a core that did not exceed 145 km in radius. On the other hand, Tethys’ shape – which resembles that of a triaxial ellipsoid – is consistent with it having a homogeneous interior (i.e. a mix of ice and rock).
This ice is also very reflective, which makes Tethys the second-brightest of the moons of Saturn, after Enceladus. There are two different regions of terrain on Tethys. One portion is ancient, with densely packed craters, while the other parts are darker and have less cratering. The surface is also marked by numerous large faults or graben.
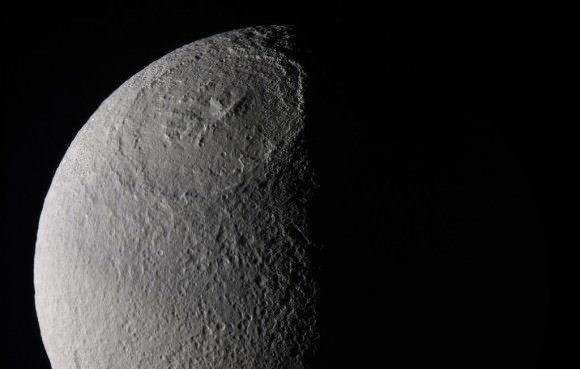
The western hemisphere of Tethys is dominated by a huge, shallow crater called Odysseus. At 400 km across, it is the largest crater on the surface, and roughly 2/5th the size of Tethys itself. Due to its position, shape, and the fact that a section in the middle is raised, this crater is also responsible for lending the moon it’s “Death Star” appearance.
The largest graben, Ithaca Chasma, is about 100 km wide and more than 2000 km long, making it the second longest valley in the Solar System. Named after the island of Ithaca in Greece, this valley runs approximately three-quarters of the way around Tethys’ circumference. It is also approximately concentric with Odysseus crater, which has led some astronomers to theorize that the two features might be related.
Scientists also think that Tethys was once internally active and that cryovolcanism led to endogenous resurfacing and surface renewal. This is due to the fact that a small part of the surface is covered by smooth plains, which are devoid of the craters and graben that cover much of the planet. The most likely explanation is that subsurface volcanoes deposited fresh material on the surface and smoothed out its features.
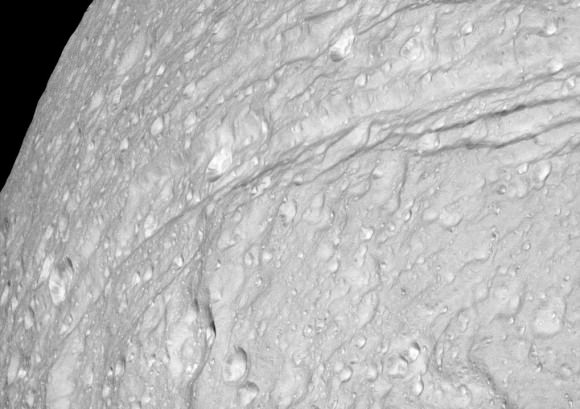
Like all other regular moons of Saturn, Tethys is believed to have formed from the Saturnian sub-nebula – a disk of gas and dust that surrounded Saturn soon after its formation. As this dust and gas coalesced, it formed Tethys and its two co-orbital moons: Telesto and Calypso. Hence why these two moons were captured into Tethys’ Lagrangian points, with one orbiting ahead of Tethys and the other following behind.
Exploration:
Tethys has been approached by several space probes in the past, including Pioneer 11 (1979), Voyager 1 (1980) and Voyager 2 (1981). Although both Voyager spacecraft took images of the surface, only those taken by Voyager 2 were of high enough resolution to truly map the surface. While Voyager 1 managed to capture an image of Ithaca Chasma, it was the Voyager 2 mission that revealed much about the surface and imaged the Odysseus crater.
Tethys has also been photographed multiple times by the Cassini orbiter since 2004. By 2014, all of the images taken by Cassini allowed for a series of enhanced-color maps that detailed the surface of the entire planet (shown below). The color and brightness of Tethys’ surface have since become sources of interest to astronomers.
On the leading hemisphere of the moon, spacecraft have found a dark bluish band spanning 20° to the south and north from the equator. The band has an elliptical shape getting narrower as it approaches the trailing hemisphere, which is similar to the one found on Mimas.
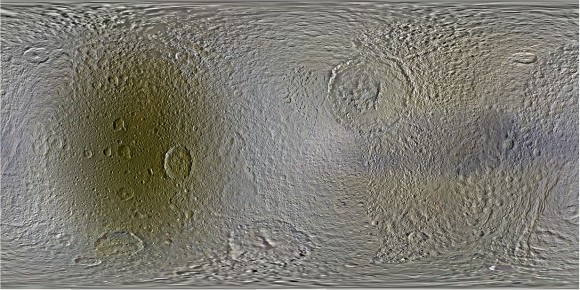
The band is likely caused by the influence of energetic electrons from Saturn’s magnetosphere, which drift in the direction opposite to the rotation of the planet and impact areas on the leading hemisphere close to the equator. Temperature maps of Tethys obtained by Cassini have shown this bluish region to be cooler at midday than surrounding areas.
At present, Tethys’ water-rich composition remains unexplained. One of the most interesting explanations proposed is that the rings and inner moons accreted from the ice-rich crust of a much larger, Titan-sized moon before it was swallowed up by Saturn. This, and other mysteries, will likely be addressed by future space probe missions.
We have many great articles about Tethys here at Universe Today. Here’s one about the story about Tethys, with a photograph taken by NASA’s Cassini spacecraft, and another about a feature on the surface of Tethys called Ithaca Chasma.
Want more info on Tethys? Check out this article from Solar Views, and this one from Nine Planets.
We have recorded two episodes of Astronomy Cast just about Saturn. The first is Episode 59: Saturn, and the second is Episode 61: Saturn’s Moons.