[/caption]
From a press release by the Subaru Telescope and the National Astronomical Observatory of Japan:
A research team led by astronomers from the University of Tokyo and the National Astronomical Observatory of Japan (NAOJ) has discovered that inclined orbits may be typical rather than rare for exoplanetary systems — those outside of our solar system. Their measurements of the angles between the axes of the star’s rotation (stellar rotational axis) and the planet’s orbit (planetary orbital axis) of exoplanets HAT-P-11b and XO-4b demonstrate that these exoplanets’ orbits are highly tilted. This is the first time that scientists have measured the angle for a small planet like HAT-P-11 b. The new findings provide important observational indicators for testing different theoretical models of how the orbits of planetary systems have evolved.
Since the discovery of the first exoplanet in 1995, scientists have identified more than 500 exoplanets, planets outside of our solar system, nearly all of which are giant planets. Most of these giant exoplanets closely orbit their host stars, unlike our solar system’s giant planets, like Jupiter, that orbit the Sun from a distance. Accepted theories propose that these giant planets originally formed from abundant planet-forming materials far from their host stars and then migrated to their current close locations. Different migration processes have been suggested to explain close-in giant exoplanets.
Disk-planet interaction models of migration focus on interactions between the planet and its protoplanetary disk, the disk from which it originally formed. Sometimes these interactions between the protoplanetary disk and the forming planet result in forces that make the planet fall toward the central star. This model predicts that the spin axis of the star and the orbital axis of the planet will be in alignment with each other.
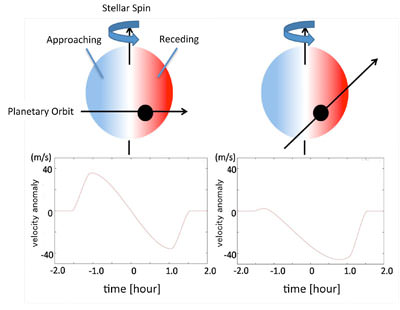
Planet-planet interaction models of migration have focused on mutual scatterings among giant planets. Migration can occur from planet scattering, when multiple planets scatter during the creation of two or more giant planets within the protoplanetary disk. While some of the planets scatter from the system, the innermost one may establish a final orbit very close to the central star. Another planet-planet interaction scenario, Kozai migration, postulates that the long-term gravitational interaction between an inner giant planet and another celestial object such as a companion star or an outer giant planet over time may alter the planet’s orbit, moving an inner planet closer to the central star. Planet-planet migration interactions, including planet-planet scattering and Kozai migration, could produce an inclined orbit between the planet and the stellar axis.
Overall, the inclination of the orbital axes of close-in planets relative to the host stars’ spin axes emerges as a very important observational basis for supporting or refuting migration models upon which theories of orbital evolution center. A research group led by astronomers from the University of Tokyo and NAOJ concentrated their observations with the Subaru Telescope on investigating these inclinations for two systems known to have planets: HAT-P-11 and XO-4. The group measured the Rossiter-McLaughlin (hereafter, RM) effect of the systems and found evidence that their orbital axes incline relative to the spin axes of their host stars.
The RM effect refers to apparent irregularities in the radial velocity or speed of a celestial object in the observer’s line of sight during planetary transits. Unlike the spectral lines that are generally symmetrical in measures of radial velocity, those with the RM effect deviate into an asymmetrical pattern (see Figure 1). Such apparent variation in radial velocity during a transit reveals the sky-projected angle between the stellar spin axis and planetary orbital axis. Subaru Telescope has participated in previous discoveries of the RM effect, which scientists have investigated for approximately thirty-five exoplanetary systems thus far.
In January 2010, a research team led by the current team’s astronomers from the University of Tokyo and the National Astronomical Observatory of Japan used the Subaru Telescope to observe the planetary system XO-4, which lies 960 light years away from Earth in the Lynx region. The system’s planet is about 1.3 times as massive as Jupiter and has a circular orbit of 4.13 days. Their detection of the RM effect showed that the orbital axis of the planet XO-4 b tilts to the spin axis of the host star. Only the Subaru Telescope has measured the RM effect for this system so far.
In May and July 2010, the current research team conducted targeted observations of the HAT-P-11 exoplanetary system, which lies 130 light years away from the Earth toward the constellation Cygnus. The Neptune-sized planet HAT-P-11 b orbits its host star in a non-circular (eccentric) orbit of 4.89 days and is among the smallest exoplanets ever discovered. Until this research, scientists had only detected the RM effect for giant planets. The detection of the RM effect for smaller-sized planets is challenging because the signal of the RM effect is proportional to the size of the planet; the smaller the transiting planet, the fainter the signal.
;The team took advantage of the enormous light-collecting power of the Subaru Telescope’s 8.2m mirror as well as the precision of its High Dispersion Spectrograph. Their observations not only resulted it the first detection of the RM effect for a smaller Neptune-sized exoplanet but also provided evidence that the orbital axis of the planet inclines to the stellar spin axis by approximately 103 degrees in the sky. A research group in the U.S. used the Keck Telescope and made independent observations of the RM effect of the same system in May and August 2010; their results were similar to those from the University of Tokyo/NAOJ team’s May and July 2010 observations.
The current team’s observations of the RM effect for the planetary systems HAT-P-11 and XO-4 have shown that they have planetary orbits highly tilted to the spin axes of their host stars. The latest observational results about these systems, including those obtained independently of the findings reported here, suggest that such highly inclined planetary orbits may commonly exist in the universe. The planet-planet scenario of migration, whether caused by planet-planet scattering or Kozai migration, rather than the planet-disk scenario could account for their migration to the present locations.
However, measurements of the RM effect for individual systems cannot decisively discriminate between the migration scenarios. Statistical analysis can help scientists determine which, if any, process of migration is responsible for the highly inclined orbits of giant planets. Since different migration models predict different distributions of the angle between the stellar axis and planetary orbit, developing a large sample of the RM effect enables scientists to support the most plausible migration process. Inclusion of the measurements of the RM effect for such a small-sized planet as HAT-P-11 b in the sample will play an important role in discussions of planetary migration scenarios.
Many research groups are planning to make observations of the RM effect with telescopes around the world. The current team and the Subaru Telescope will play an integral role in investigations to come. Continuous observations of transiting exoplanetary systems will contribute to an understanding of the formation and migration history of planetary systems in the near future.
So how did we go from having measured two systems with known sample bias (close in, very likely migrated planets) to the title of this article “Most Exoplanetary Solar Systems Have Inclined Orbits”? I think a “may” have dropped out, as in “_may_ have”.
And then we could argue that most solar like systems, likely with a late Jupiter-Saturn et cetera Nice model migration against some asteroid belt, may have non-inclined orbits; because there we know 1 sample, again consisting of all such measured. (Assuming the article observations makes out the rest.) Which could be natural I guess, if belts are sufficiently “protoplanetary disk” like?
Interesting. The same principle works for triple stars, where the third component preferentially inclined to the orbit of the two inner or closer binary. It has been proven that theoretically, looking at the relative inclinations that the stability of the so-called ‘ξ-value’ should favour the relative inclination around 45° to 50°. If an observed star was found which favours the parallel or perpendicular position at 0° or 180°, as it would cause rapid changes in the general orbital parameters.
Charles Worley in 1968 found that due to these observed relative inclinations, in respect to the Earth, it is more probable that the Earth will be placed in the acute angle rather than the obtuse angle. Analysis shows within the population of multiples, which might also apply to extra-planetary systems, that the total number of apparent counter-revolving systems becomes about 31%.
This is the so-called co-planarity of triples ‘problem’, and this is based on whether the orbits are either co-revolving or counter revolving. Importantly this may
limits the stability of multiples can be partly dependent on the direction of revolution of the primary or inner pair.
On the other hand this is not observed in the solar system, which might be explained by the complex perturbations between many planets; though the long-term stability of the inner planets may vary wildly over tens of millions of years.
Some of this early work on multiple may in the end apply to extra-solar planetary systems. Like many things in astronomy, we need more data.