[/caption]
I feel a certain empathy for brown dwarfs. The first confirmed finding of one was only fifteen years ago and they remain frequently overlooked in most significant astronomical surveys. I mean OK, they can only (stifles laughter) burn deuterium but that’s something, isn’t it?
It has been suggested that a clever way of finding more brown dwarfs is in the radio spectrum. A brown dwarf with a strong magnetic field and a modicum of stellar wind should produce an electron cyclotron maser. Roughly speaking (something you can always depend on from this writer), electrons caught in a magnetic field are spun energetically in a tight circle, stimulating the emission of microwaves in a particular plane from the star’s polar regions. So you get a maser, essentially the microwave version of a laser, that would be visible on Earth – if we are in line of sight of it.
While the maser effect can probably be weakly generated by isolated brown dwarfs, it’s more likely we will detect one in binary association with a less mass-challenged star that is capable of generating a more vigorous stellar wind to interact with the brown dwarf’s magnetic field.
This maser effect is also proposed to offer a clever way of finding exoplanets. An exoplanet could easily outshine its host star in the radio spectrum if its magnetic field is powerful enough.
So far, searches for confirmed radio emissions from brown dwarfs or orbiting bodies around other stars have been unsuccessful, but this may become achievable in the near future with the steadily growing resolution of the European LOw Frequency ARray (LOFAR), which will be the best such instrument around until the Square Kilometer Array (SKA) is built – which won’t be seeing first light before at least 2017.
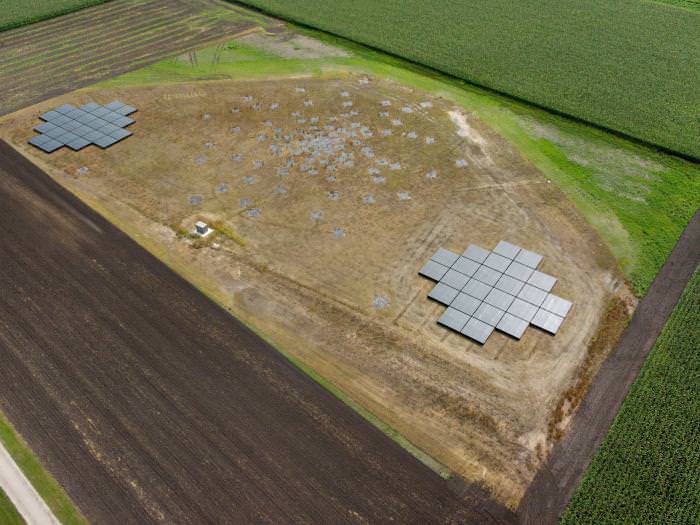
But even if we can’t see brown dwarfs and exoplanets in radio yet, we can start developing profiles of likely candidates. Christensen and others have derived a magnetic scaling relationship for small scale celestial objects, which delivers predictions that fit well with observations of solar system planets and low mass main sequence stars in the K and M spectral classes (remembering the spectral class mantra Old Backyard Astronomers Feel Good Knowing Mnemonics).
Using the Christensen model, it’s thought that brown dwarfs of about 70 Jupiter masses may have magnetic fields in the order of several kilo-Gauss in their first hundred million years of life, as they burn deuterium and spin fast. However, as they age, their magnetic field is likely to weaken as deuterium burning and spin rate declines.
Brown dwarfs with declining deuterium burning (due to age or smaller starting mass) may have magnetic fields similar to giant exoplanets, anywhere from 100 Gauss up to 1 kilo-Gauss. Mind you, that’s just for young exoplanets – the magnetic fields of exoplanets also evolve over time, such that their magnetic field strength may decrease by a factor of ten over 10 billion years.
In any case, Reiners and Christensen estimate that radio light from known exoplanets within 65 light years will emit at wavelengths that can make it through Earth’s ionosphere – so with the right ground-based equipment (i.e. a completed LOFAR or a SKA) we should be able to start spotting brown dwarfs and exoplanets aplenty.
Further reading: Reiners, A. and Christensen, U.R. (2010) A magnetic field evolution scenario for brown dwarfs and giant planets.
“…Old Backyard Astronomers Feel Good Knowing Mnemonics…” oTay… I hadn’t heard that one before. I use the less politically correct – “Oh, Be A Fine Girl (and) Kiss Me” ~@; )
LOFAR and SKA telescopes concepts are kinda cool… I hope we can spread the baseline to Luna some day! Then perhaps an NEO~
This was cool but where is Planet X when we need it? π
tell me if I have this wrong – the distribution of objects in our galaxy (or at least in this spiral arm) is less for the Big Ones and More for the small ones. Given that principle, we there should be more brown dwarves than their are stars but less than planets.
And given the discovery of chemosynthetic life , one wonders if there could be a civilization one a world about a brown dwarfs.
Could be interesting!
@ Vanamonde
I think your ‘distribution of objects’ comment is broadly consistent with current data – though it’s a bit early to be sure about the prevalence of planets around other stars. We know that one star has eight planets – but otherwise we are still counting π
The chances of life or civilisation appearing on a world in orbit around a brown dwarf seem remote. BDs may only radiate strongly for the first hundred million years or so after birth – so that’s not much time to play with.
Red dwarfs (the most numerous ‘real’ stars) are better candidates – given they can burn for many billions of years. There’s a detailed wikipedia entry on the issue here:
http://en.wikipedia.org/wiki/Habitability_of_red_dwarf_systems
Thanks, this was another gem! I didn’t know about the generality of heat flux dependence of the internal dynamo over such a variety of objects. It certainly shore up the current Earth dynamo model (once again).
Or rather two gems, as the press release suggest magnetic field detection is another method of localizing exoplanets. An attractive proposal IMHO.
Nitpicking in the rough: a projected circle, an electron gyro-trajectory is generally a helix.
Well, I think the Kepler 1st release gave enough statistics to tentatively predict this:
“Small candidate planets with periods less than 30 days are much more common than large candidate planets with periods less than 30 days and that the ground-based discoveries are sampling the large-size tail of the size distribution. Note that for a substantial range of planet sizes, a 1/R2 curve fits the Kepler data well. Assuming the false positive rate and other biases discussed above are independent of planet size for planets larger than two Earth radii, this implies that the frequency of planets decreases with the area of the planet.”
Of course this needs to be extended to larger orbital periods, but considering the prevalence of migrated hot Jupiters that could mess (or rather help!) with this statistic it could be a robust result.
Let me try some handwaving: the 1/r^2 curve could perhaps be understood as the ability to scope up mass from the protoplanetary disk, which will be proportional to the cross sectional area of the growing planet. Smaller planets means many more can aggregate out of the same disk volume before it starts to scatter too much. (I wonder what simulations geared against the Kepler data say?!)
So for life to start we need many many many years.
So what if a planet is very very close, so close to a star that one year would be equal of one Earth day.
This way life could start 365 times faster than earth. Creation of life would require only a few 10.000 years.
Yes I am joking! Yes I am joking! I just couldn’t resist.
I wrote elsewhere a couple of weeks ago about dwarf stars in connection with dark energy and de Sitter spacetime. I commented that it was somewhat remarkable that we live at just a time where we now observe the onset of the exponential expansion of the universe. Observers or intelligent life that might have come about 3-4 billion years ago would have observed the universe in a matter dominated phase and have had difficulty detecting this vacuum effect. Observers which might come about 10-20 billion years from now would have a difficult time seeing much beyond the galaxy, as the rest of universe redshifts off to z – -> infinity. So we seem to exist at some optimal time in the evolution of the universe. I then made the comment that 20 billion years from now the galaxy will consist almost entirely of red dwarf stars, which make poor stellar sources of bio-planets. So I suggested that astronomers are not likely 20 billion years from now.
I think the best prospect for a bio-planet around an M class star is if the planet is a double planet. So an Earth sized planet in a close orbit with a moon to Mars sized body would not get tidally locked to the star in a close orbit. Of course there are lots of other questions, such as the fact the blackbody radiation peak for M-class stars is in the 550nm-1000nm range (red to IR), which is not optimal for photosynthesis. So the process of extracting energy might have analogues with the thermal energy sulfur process seen with life around ocean vents or βsmokers.β So there is some prospect for life on planets around some of the more robust M-class stars
Maybe if there persists self organizing systems in the universe, say if humanity ends up lofting von Neumann probes into the galaxy that evolve and persist for billions of years, that these might in 20 billion years set up Dyson-like spheres around M class stars The galaxy might then contain an information-net of systems around red dwarf stars that persists for over 100 billion years. Of course this is pure speculation, but is not impossible.
K-class stars are a better prospect for life. There are more of these than G-class stars and have a bit more energy behind them than red dwarf stars do. A K class star with .8M_{sol} could sustain a planet at about .5AU with conditions similar to Earth for a very long time without tidal locking issues.
This search is interesting, and it might be useful for looking in at black holes in galaxy centers. A black hole that is quiet would still command the magnetohydrodynamics of a diffuse gas-plasma with a magnetic field. There could then be significant maser physics going on.
Cheers LC
Lawrence,
it occurs to me that the habitability of the universe is a function of time. As the universe ages, it produces more and more useful elements like Carbon and Oxygen. These are not only necessary for life because living things are made of them, but because they seem to allow the creation of smallish stars like the Sun. But sooner or later there will come a time when the universe is dominated by little less-useful red dwarfs and brown dwarfs. So I suspect that there will be a time when the life-friendliness of the universe will hit a peak, and I also suspect we are currently at or near that peak now. If this suspicion is correct, the it’s probably just coincidence that this peak happens to coincide with the time when the exponential expansion of the universe is just becoming noticeable.
Nexus
It might be at this peak now, unless my loose speculation about slef replicating and evolving von Neumann probes or nanobots takes hold. We seem to be at a phase where an observer gets the optimal amount of information about the universe as well.
LC
Slightly OT, but this:
Neither a coincidence nor remarkable, but a natural result of that the likelihood for observers are maximized at this era over the whole cosmological landscape:
“Typical observers find themselves in a flat universe, at the onset of vacuum domination, surrounded by a recently produced bath of relativistic quanta. These quanta are neither very dilute nor condensed, and thus appear as a roughly thermal background. Their characteristic wavelength is of order the inverse fourth root of the vacuum energy.
These predictions hold for completely arbitrary observers, in arbitrary vacua with potentially exotic particle physics and cosmology.
They agree with observation: We live in a flat universe at the onset of vacuum domination, whose dominant entropy production process (the glow of galactic dust) has recently produced a radiation bath (the cosmic infrared background). This radiation is marginally dilute, relativistic, and has a wavelength of order 100 microns, as predicted.” [Bousso et al., 2010; my bold.]
It’s like religious finetuning; you may think it is a low probability but finding out the actual (and relevant) likelihood paints a different picture.
Really, this has been known, oh, already since the beginning of 2010. What do they learn about environmental principles in cosmology these days? Oh, and get off my lawn! π
Oh, I forgot; this result is of course only applicable if there is an inflationary and/or string cosmological landscape at all.
[But if there isn’t, it would be slightly remarkable in itself as it goes against a lot of natural properties of physics akin to our beloved fields et cetera, and further leave these observations as exactly remarkable coincidences. Personally I won’t bet on that for the moment.]
I wonder if we should be invoking ‘vacuum domination’ as though it means something definitive.
The predictions of quantum mechanical ‘vacuum energy” are out by 120 orders of magnitude from anything we can observe in the universe at a macro-scale.
Agreed, we’ve just discovered the universe is undergoing accelerated expansion. But suggesting that the cause of that is an injection of lots of extra vacuum into the system seems like a big intellectual leap.
If you want to include Brown Dwarfs in a mnemonic:
Oh Be A Fine Girl (and) Kiss Me Lovingly Tonight.
Maybe someone could use that as a pickup line.
Torbjorn Larsson OM: This takes us a bit astray from brown dwarfs, but… . I read this paper by Bousso and Harnik earlier this year. Bousso has found a cosmological generalization of the Bekenstein bound, called the Bousso bound. These bounds determine the maximum entropy for a system with some length scale. For a black hole this is S = k A/4L_p, k = Boltzmann constant, A = 4pi R^2 the area of the black hole horizon and L_p the Planck length. This rule associates entropy of a system with some volume according to the size of the bounding region with one dimension smaller. This extends to higher dimensions as well. The Anti de Sitter group with two time dimensions SO(4,2) ~ SU(2,2), or the space AdS_{n+1}, then has a boundary which determines the conformal completion of the AdS which is an Einstein spacetime E_n that has the same content as an n-sphere. This Einstein space is a de Sitter spacetime which is conformally equivalent to a flat spacetime. For n = 5 the S^5 sphere is a conformal field theory, which is equivalent to the data on the conformal boundary of the AdS. This sets up the universe as determined by a horizon induced entropy.
However, something is a bit odd, for the inflationary spacetime of the universe has a cosmological constant /\ = 10^{110} times the value of today, or about 13 orders of magnitude smaller than the 10^{123} value for the Planck energy density. The value of the vacuum energy is determined by a potential function over a scalar field. The potential is nearly constant for some value of the field so as to permit 62-63 efolds of the space — e^{63} expansion of the space in inflation. The de Sitter space exponentially expands to this limit and then stops where the potential function becomes a quadratic function of the scalar field, called the inflaton field. The vacuum energy density is then rapidly reduced to the value we find today and the energy gap produced generates the mass-energy of the universe.
As to what set this up is in the domain of observational uncertainty. It is intellectually satisfying to think the universe emerged from some quantum fluctuation or tunneling out of a vacuum state or of some vacuum energy (say near a black hole singularity) from some other universe.
Now everything seems good, but there are some fine tuning questions here that are troublesome. In particular, why did the universe have this number of e-folds, for without this number (62-63) the universe would be too dense or diffuse to permit galaxy formation. You either get black holes or a diffuse gas. This then sets up the conditions we see today, with the amount of mass-energy that gravitates and the vacuum energy density which induces dark energy or so called eternal inflation. In this paper the authors discuss the βcausal diamond,β which is how they use Bousso bound to connect the de Sitter inflationary universe to the FLRW radiation and matter dominated universe, which is then connected to the latent de Sitter βvacuum dominatedβ universe we observe today. In the end these constructions rely upon the landscape philosophy. In this there is a large space of sorts for that inflaton field with slopes and valleys, and the cosmos we observe is due to a local outcome of this field.
It does all lead back to what I see as the remarkable observation that we exist at a time where we observe the onset of dark energy dominated phase, but can look back far enough to see the end of the radiation dominated phase. The Planck spacecraft may find B-modes in the CMB, which are signatures of the early inflationary period. This would contrast with intelligent life that might have emerged prior to 4 billion years ago, who would not observe the dark energy phase easily. Into the future beyond 10 billion years intelligent observers will see the CMB redshifted far into the long radio band, and distant galaxies redshifted beyond the optical and IR EM spectra. These observers would conclude, as we did earlier prior to Hubble, that their galaxy is an βisland universe.β Yet such observers would find it hard to falsify this conclusion, and much beyond 10 billion years in the future the exponential expansion of the universe would make this far more difficult.
So this leads to dwarf stars, in this round about way. This galaxy, as with all others on the Hubble frame of comoving time, will beyond 10 billion years consist almost entirely of red dwarf stars. Stars such as the sun will be a highly energetic oddity — sort of how we think of stars such as Spica or the Pistol star. If stars today are popI stars, then stars then would be pop0 stars, and then pop(-I) and so forth. All the time stars will become more dwarfish, weaker and redder. So we might then ask the question of whether there can emerge intelligent life around a red dwarf star. If this happens then it means in the next 10 to 100 billion years there will be intelligent observers who may get a false picture of the universe. Or at least they will observe a universe and construct models of it that are more false than what we derive. So the question of whether bio-planets can exist around such stars is somewhat interesting, and might have some relevance to the so called anthropic principle with respect to cosmology.
LC
@ LBC
Thanks for your thoughtful response. I was seeking to suggest that vacuum energy (measurable on a micro scale) doesn’t really help to explain accelerated expansion measurable at a cosmic scale – nor how a de Sitter universe works.
Anyhow, I agree it’s off topic π
@Dark Gnat
Often banal astronomy facts gradually kill my leisure time π
Many brown dwarfs are associated with red dwarf stars. Maybe the detection of coherent microwave radiation from red dwarf stars would reveal the frequency of red dwarf stars with brown dwarfs. Maybe some of these brown dwarf stars have βmoonsβ or βplanets,β I am not sure what these would be called, and a few of these satellites might then be biologically active. This would prevent the tidal locking problem, which I think is fatal for any prospect for life on a terrestrial planet which might orbit a red dwarf.
The number of possible configurations in the universe is almost unbounded, and it might then be there would be biologically active planets in systems similar to this. A few might give rise to intelligent life. One thing which such beings would observe is there day time sky would be black, but with the bright star. Red dwarf stars have black body distributions that peak in the red to IR and produce little blue light. Bye bye blue sky! ETI around such a planet now would observe the same universe we do. However, beyond 10 billion years from now the universe would appear very differently. They would see the galaxy as the βisland universe.β Maybe if some ETI are enterprising enough they might try to observe far out in long wavelength EM radiation and observe other galaxies in the radio spectrum and find the CMB in the very long wavelength band. Yet if such ETI emerge 100 billion years from now on a red dwarf system I think their cosmic understanding would be hopelessly blocked.
Maybe it is not that different from our situation. For various reasons I think our ability to observe signatures of the universe on more fundamental levels will become ever more oblique and uncertain. Yet our situation is clearly better than astronomers will find > 10 billion years from now.
LC
It is unfortunate the Ionosphere limits the ability to 65LY. I suppose this is good and bad, allowing us to limit ourselves to the nearest possibilities, however it really cuts down on the probability of finding exoplanets which may have life.
Perhaps in the near future, we can look at building a constellation of satellites in space… each at certain legrange points which will increase our range.
brown dwarfs are up to 70 jupiter masses, and are the most common near stellar objects held by electron degeneracy forces lacking sufficient mass to form a star. they may have many tiny far icy moons, even liquid planets close to infrared heat are awaiting discovery with better telescopes. Planets need both a solid uprising mountain building or tectonic outer crust of land, above sea level makes for better chance of life, and Mars had both 4 billion years ago and is ~ 1/5 the mass of earth. extremely likely that at least a few brown dwarfs have produced survivable intelligent life with space travel technology. The stars allow 30 million years with a strong magnetic field to shield from supernova radiation. After that, they cool down and weaken in magnetic intensity against cosmic radiation. Our sun is not so common and becomes special, when compared to the abundance of brown and red dwarf stars. life using both visible and infrared light exists on earth.
Brown dwarf stars are not held up by degenerate electron pressure. That is with white dwarf stars, which are collapsed stars less than 1.4 times the mass of the sun. These stars collapse into a body about the size of the Earth with very high densities. Brown dwarf stars are held up by more ordinary electrostatic repulsions between atoms.
LC
Thank you, Mr. Crowell for understanding that I am talking mainly about ecologies without steller input. But would thermal vents in deep ocean rifts but fueled only by tidal effects? I do not believe the ones we have actually discovered (like all over the oceans) are.
And what about a planet larger than the earth but smaller than Neptune. Might it provide enough heat for even surface life to evolve?
In the fact of lack of evidence, we have no way of knowing, but we are just playing with speculations here. I believe these are possibilies.
The curious thing about planets is we are at a stage where we are observing them and getting better information about them. Prior to 1993 we had no knowledge of them. What I find interesting is how the diversity of forms these extrasolar planets, and planetary systems, take is far beyond what our prior conjectures imagined.
We may get some close ups of planets within a few tens of light years, maybe within a hundred light years before very long. If all progresses with space science I see little reason why we could not send probes to stars within 10-25 light years before the middle or end of this century.
LC