Well, here’s a bit of a first for AWAT, because this is a story about a telescope. But it’s not your average telescope, being composed of a huge chunk of Antarctic ice with a very large cosmic ray muon filter attached to the back of it, which is called the Earth.
Commenced in 2005, the IceCube Neutrino Observatory is now approaching completion with recent installation of a key component DeepCore. With DeepCore, the Antarctic observatory is now able to observe the southern sky, as well as the northern sky.
Neutrinos have no charge and are weakly interactive with other kinds of matter, making them difficult to detect. The method employed by IceCube and by many other neutrino detectors is to look for Cherenkov radiation which, in the context of IceCube, is emitted when a neutrino interacts with an ice atom creating a highly energized charged particle, such as an electron or a muon – which shoots off at a speed greater than the speed of light, at least greater than the speed of light in ice.
The advantage of using Antarctic ice as a neutrino detector is that it is available in large volumes and thousands of years of sedimentary compression has squeezed most impurities out of it, making it a very dense, consistent and transparent medium. So, not only can you see the little flashes of Cherenkov radiation, but you can also make reliable predictions about the trajectory and energy level of the neutrino which caused each little flash.
The structure of IceCube incorporates strings of evenly spaced basketball-sized Cherenkov detectors lowered into the ice through drill holes to depths of nearly 2.5 kilometers. The DeepCore component is a more compact array of detectors, positioned in the clearest ice deep within IceCube, designed to enhance the sensitivity of IceCube for neutrino energies less than 1 TeV.
Prior to DeepCore being finished, it was only feasible to accurately measure the effects of upwardly moving neutrinos – that is, neutrinos that had already passed through the Earth and, if of a cosmic origin, had actually come from the northern sky. Any downwardly moving neutrinos from the southern sky were lost in noise created by cosmic ray muons which are able to penetrate IceCube, creating their own Cherenkov radiation without neutrinos being involved.
However, with the greater sensitivity offered by DeepCore, coupled with IceTop, which is a set of surface level Cherenkov detectors able to differentiate external muons entering from the surface, it is now possible for IceCube to make neutrino observations of the southern sky as well.
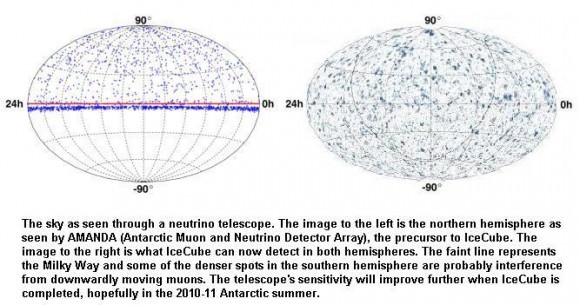
IceCube’s key scientific goal is to identify neutrino point sources in the sky, which may include supernova and gamma ray bursts. Neutrinos are speculated to account for 99% of the energy release of a Type 2 supernova – suggesting that we may be missing a lot of information when we just focus on the electromagnetic radiation that is emitted.
It is also speculated that IceCube might provide indirect evidence of dark matter. The thinking is that if some dark matter was caught in the centre of the Sun, it would be annihilated by the extreme gravitational compression present there. Such an event should produce a sudden burst of high energy neutrinos, independent of the normal neutrino output resulting from solar fusion reactions. That’s a long chain of suppositions to gain indirect evidence of something, but we’ll see.
Interesting article! The relevant paper (PDF) with an illustration of the “IceCube” is available here: “Kilometer-Scale Neutrino Detectors: First Light“.
Thanks for the article and thanks for the reference! Slightly OT, I like when astronomy forces you to use “exa” (as in EeV in the ref). Even if the planet-to-star ratio is a mere 10^-4, there are exaquantities of exoplanets in the visible universe.
Question, and a possible nitpick: “annihilated by the extreme gravitational compression”. That is some funny particle that annihilates from gravity outside black holes?!
The reference gives the to me more plausible model of particles being “gravitationally trapped” (Sun especially) and then annihilated. The reference given explains that it is sometime weak interaction elastic scatter with nuclei of gravitationally bound matter that does the trapping. Gotta get rid of that momentum excess!
I believe WIMPs can be their own antiparticles in some models. (Easier said than done, I guess, for example that would preclude them being everyday fermions. Right, they _aren’t_ everyday fermions anyway…)
Anyway they are claimed to see an enhanced annihilation rate if trapped to higher density in gravitational wells, possibly balancing trapping with annihilation (and evaporation). At least that is how I briefly read the link.
Would it be possible to stick a sister telescope in Greenland, or is that inland ice just not clear and thick enough?
And if it were, could we build a com-link out of them?
@ Torbjorn Larsson OM
Fair nitpick. I recall the full explanation from the article, but went for brevity. Presumably, it’s not just the heat of the Sun’s core that does the annihilation since similar temperatures are present in the corona.
So, the implication is that cramming lots of matter together at very high densities can annihilate any dark matter that is present. Annihilate was verbatim, I think ‘break down’ or ‘transform’ might be more accurate descriptions of this highly speculative process?
This appears similar to the result by Ahlawalia on neutrino oscillations in gravity fields. The CKM matrix for the oscillations is boosted by the local gravity fields so that e, mu and tau neutrinos have different recurrences.
LC