In 2017, astronomers detected gravitational waves from colliding neutron stars for the first time: a kilonova. Enormous amounts of heavy metals were detected in the light from the explosion, and astronomers continued to watch the expanding debris cloud.
Researchers have continued to study this event. Now, using a three-dimensional computer simulation, they have created a new recreation of this merger — second by second, as it happened — giving insights into all the high-energy mayhem and heavy elements formation in this catastrophic event.
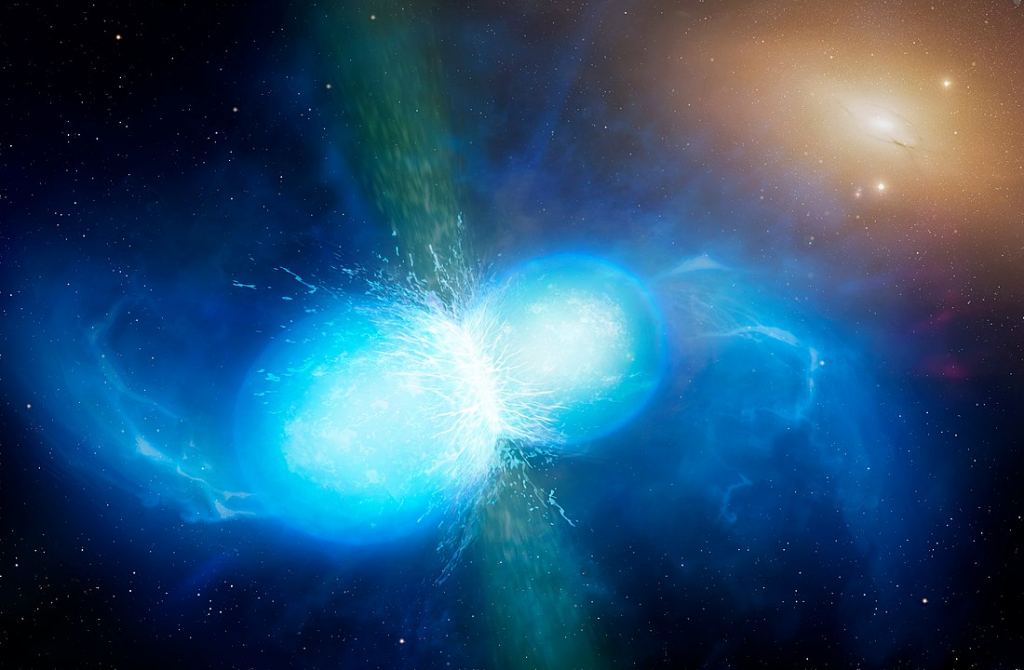
“The unprecedented agreement between our simulations and the observation of kilonova AT2017gfo [also known as GW170817] indicates that we understand broadly what has taken place in the explosion and aftermath,” said Luke J. Shingles, scientist at GSI Helmholtz Centre for Heavy Ion Research and the Facility for Antiproton and Ion Research (GSI/FAIR) in Germany. Shingles is the lead author of a new paper in published in Astrophysical Journal Letters.
Neutron stars are the crushed, leftover cores of massive stars that long ago exploded as supernovas. The merging stars likely had masses between 10 and 60 percent greater than that of our Sun, but they were no wider than an average-sized city. The pair whirled around each other hundreds of times a second. As they drew closer and orbited faster, the stars eventually broke apart and merged, producing both a gamma-ray burst and a rarely seen explosion called a kilonova.
This event was observed on August 17, 2017 by the LIGO–Virgo gravitational wave facilities. Several telescopes watched the aftermath of this event including the space telescopes Swift, Hubble, Chandra and Spitzer missions, along with dozens of ground-based observatories. The Pan-STARRS survey was able to capture the fading glow of the blast’s expanding debris.
At a press briefing shortly after the detection of this event — observed both in graviational waves and light — researcher Edo Berger, from the Harvard Smithsonian Center for Astrophysics said, “These observations reveal the direct fingerprints of the heaviest elements in the periodic table. “The collision of the two neutron stars produced 10 times of mass of Earth in gold and platinum alone. Think about how as these materials are flying out of this event, they eventually combine with other elements to form stars, planets, life … and jewelry.”
The data from all the observatories now have allowed the researchers to produce, for the first time, a three-dimensional simulation that recreates the neutron-star merger and the subsequent radiative transfer with tens of millions of atomic transitions of heavy elements.
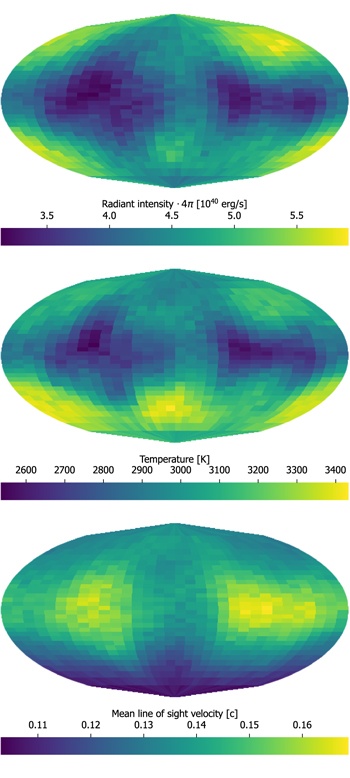
The researchers said that with a 3D model, the observed light can be predicted for any viewing direction. For example, when viewed nearly perpendicular to the orbital plane of the two neutron stars (as observational evidence indicates for the kilonova AT2017gfo) the model predicts a sequence of spectral distributions that look remarkably like the observations for AT2017gfo.
“Research in this area will help us to understand the origins of elements heavier than iron (such as platinum and gold) that were mainly produced by the rapid neutron capture process in neutron star mergers,” said Shingles in a GSI/FAIR press release.
In their paper, the team said about half of the elements heavier than iron are produced during an event like a kilonova, within the environment of extreme temperatures and neutron densities. The explosion ejects matter with just the right conditions to “produce unstable neutron-rich heavy nuclei by a sequence of neutron captures and beta-decays. These nuclei decay to stability, liberating energy that powers an explosive ‘kilonova’ transient, a bright emission of light that rapidly fades in about a week.”
The researchers said their 3D simulation combines several areas of physics, including the behavior of matter at high densities, the properties of unstable heavy nuclei, and atom-light interactions of heavy elements.
With further research, they hope to increase the precision with which they can predict and understand features in the spectra, which will further our understanding of the conditions in which heavy elements are synthesized.