Like most galaxies, our Milky Way has a dark monster in its middle: an enormous black hole with the mass of 4 million Suns inexorably dragging in anything that comes near. But even at this scale, a supermassive black hole like Sgr A* doesn’t actually consume everything that it gets its gravitational claws on — thanks to the Chandra X-ray Observatory, we now know that our SMB is a sloppy eater and most of the material it pulls in gets spit right back out into space.
(Perhaps it should be called the Cookie Monster in the middle.*)
New Chandra images of supermassive black hole Sagittarius A*, located about 26,000 light-years from Earth, indicate that less than 1% of the gas initially within its gravitational grasp ever reaches the event horizon. Instead, much of the gas is ejected before it gets near the event horizon and has a chance to brighten in x-ray emissions.
The new findings are the result of one of the longest campaigns ever performed with Chandra, with observations made over 5 weeks’ time in 2012.
Read more: Chandra Stares Deep into the Heart of Sagittarius A*
“This new Chandra image is one of the coolest I’ve ever seen,” said study co-author Sera Markoff of the University of Amsterdam in the Netherlands. “We’re watching Sgr A* capture hot gas ejected by nearby stars, and funnel it in towards its event horizon.”
As it turns out, the wholesale ejection of gas is necessary for our resident supermassive black hole to capture any at all. It’s a physics trade-off.
“Most of the gas must be thrown out so that a small amount can reach the black hole”, said co-author Feng Yuan of Shanghai Astronomical Observatory in China. “Contrary to what some people think, black holes do not actually devour everything that’s pulled towards them. Sgr A* is apparently finding much of its food hard to swallow.”
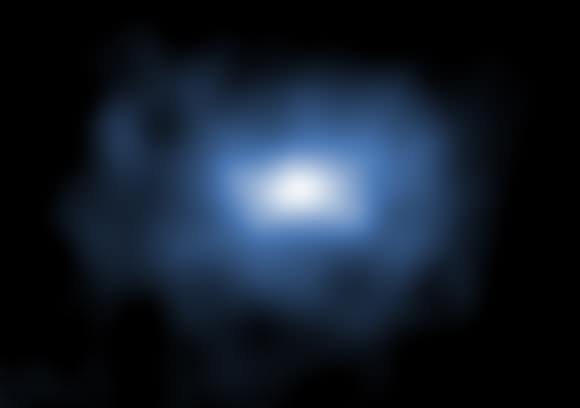
If it seems odd that such a massive black hole would have problems slurping up gas, there are a couple of reasons for this.
One is pure Newtonian physics: to plunge over the event horizon, material captured — and subsequently accelerated — by a black hole must first lose heat and momentum. The ejection of the majority of matter allows this to occur.
The other is the nature of the environment in the black hole’s vicinity. The gas available to Sgr A* is very diffuse and super-hot, so it is hard for the black hole to capture and swallow it. Other more x-ray-bright black holes that power quasars and produce huge amounts of radiation have much cooler and denser gas reservoirs.
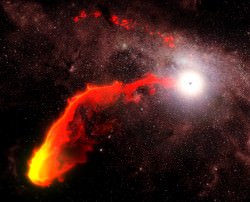
Located relatively nearby, Sgr A* offers scientists an unprecedented view of the feeding behaviors of such an exotic astronomical object. Currently a gas cloud several times the mass of Earth, first spotted in 2011, is moving closer and closer to Sgr A* and is expected to be ripped apart and partially consumed in the coming weeks. Astronomers are eagerly awaiting the results.
“Sgr A* is one of very few black holes close enough for us to actually witness this process,” said Q. Daniel Wang of the University of Massachusetts at Amherst, who led the study.
Watch Black Holes: Monsters of the Cosmos
Source: Chandra press release. Read the team’s paper here.
Image credits: X-ray: NASA/UMass/D.Wang et al., IR: NASA/STScI
_________________
*Any resemblance of Sgr A* to an actual Muppet, real or fictitious, is purely coincidental.