In this series we are exploring the weird and wonderful world of astronomy jargon! You probably don’t know how close you are to today’s topic: parallax!
Continue reading “Astronomy Jargon 101: Parallax”Astronomy Jargon 101: Parallax
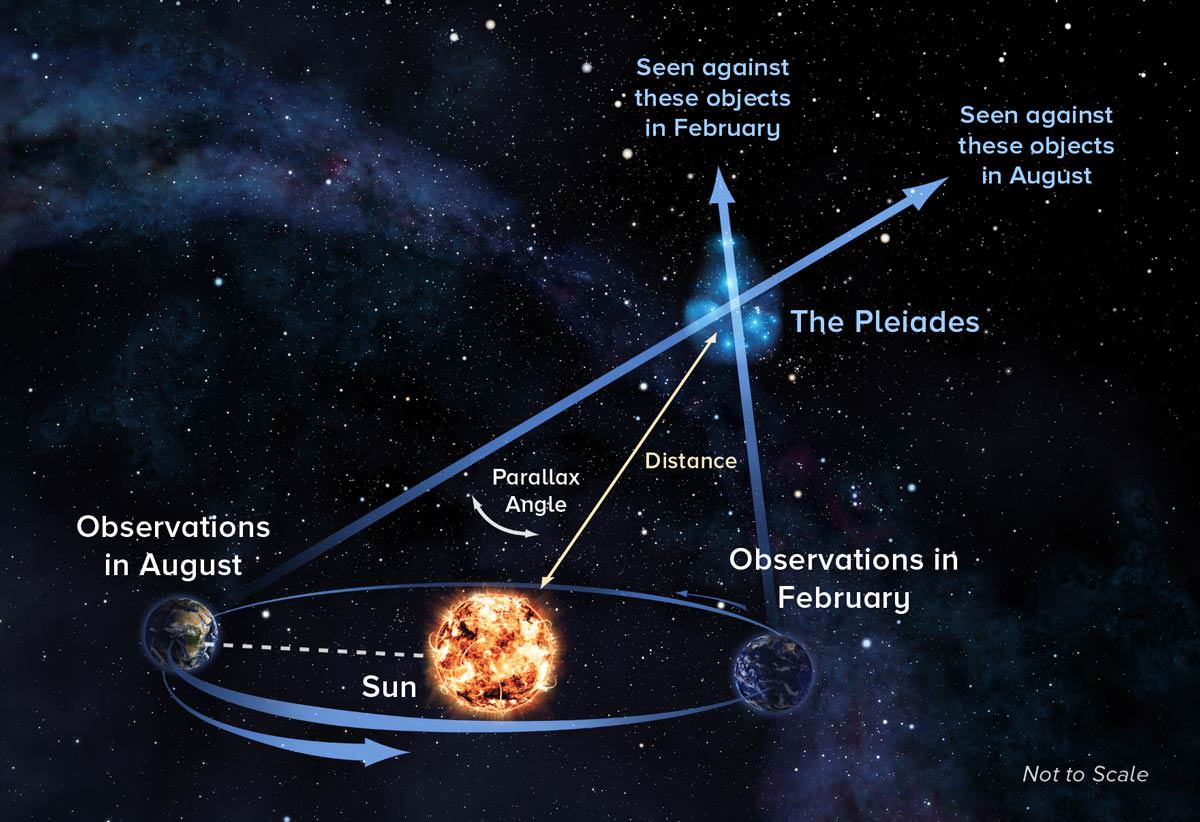
In this series we are exploring the weird and wonderful world of astronomy jargon! You probably don’t know how close you are to today’s topic: parallax!
Continue reading “Astronomy Jargon 101: Parallax”Binary star systems are everywhere. They make up a huge percentage of all known solar systems: from what we can tell, about half of all Sun-like stars have a binary partner. But we haven’t really had a chance to study them in detail yet. That’s about to change. Using data from the European Space Agency’s Gaia spacecraft, a research team has just compiled a gigantic new catalog of nearby binary star systems, and it shows that at least 1.3 million of them exist within 3000 light-years of Earth.
Continue reading “Our Part of the Galaxy is Packed with Binary Stars”In 1964 two Aerobee suborbital rockets were launched with the goal of mapping x-ray sources in the sky. Each rocket contained a directed Geiger counter, so that as the rocket rotated at the peak of its trajectory to measure the direction of x-ray sources. The project discovered eight x-ray sources, including a particularly bright one in the constellation Cygnus. It became known as Cygnus X-1.
The night sky, is the night sky, is the night sky. The constellations you learned as a child are the same constellations that you see today. Ancient people recognized these same constellations. Oh sure, they might not have had the same name for it, but essentially, we see what they saw.
But when you see animations of galaxies, especially as they come together and collide, you see the stars buzzing around like angry bees. We know that the stars can have motions, and yet, we don’t see them moving?
How fast are they moving, and will we ever be able to tell?
Stars, of course, do move. It’s just that the distances are so great that it’s very difficult to tell. But astronomers have been studying their position for thousands of years. Tracking the position and movements of the stars is known as astrometry.
We trace the history of astrometry back to 190 BC, when the ancient Greek astronomer Hipparchus first created a catalog of the 850 brightest stars in the sky and their position. His student Ptolemy followed up with his own observations of the night sky, creating his important document: the Almagest.
In the Almagest, Ptolemy laid out his theory for an Earth-centric Universe, with the Moon, Sun, planets and stars in concentric crystal spheres that rotated around the planet. He was wrong about the Universe, of course, but his charts and tables were incredibly accurate, measuring the brightness and location of more than 1,000 stars.
A thousand years later, the Arabic astronomer Abd al-Rahman al-Sufi completed an even more detailed measurement of the sky using an astrolabe.
One of the most famous astronomers in history was the Danish Tycho Brahe. He was renowned for his ability to measure the position of stars, and built incredibly precise instruments for the time to do the job. He measured the positions of stars to within 15 to 35 arcseconds of accuracy. Just for comparison, a human hair, held 10 meters away is an arcsecond wide.
Also, I’m required to inform you that Brahe had a fake nose. He lost his in a duel, but had a brass replacement made.
In 1807, Friedrich Bessel was the first astronomer to measure the distance to a nearby star 61 Cygni. He used the technique of parallax, by measuring the angle to the star when the Earth was on one side of the Sun, and then measuring it again 6 months later when the Earth was on the other side.
Over the course of this period, this relatively closer star moves slightly back and forth against the more distant background of the galaxy.
And over the next two centuries, other astronomers further refined this technique, getting better and better at figuring out the distance and motions of stars.
But to really track the positions and motions of stars, we needed to go to space. In 1989, the European Space Agency launched their Hipparcos mission, named after the Greek astronomer we talked about earlier. Its job was to measure the position and motion of the nearby stars in the Milky Way. Over the course of its mission, Hipparcos accurately measured 118,000 stars, and provided rough calculations for another 2 million stars.
That was useful, and astronomers have relied on it ever since, but something better has arrived, and its name is Gaia.
Launched in December 2013, the European Space Agency’s Gaia in is in the process of mapping out a billion stars in the Milky Way. That’s billion, with a B, and accounts for about 1% of the stars in the galaxy. The spacecraft will track the motion of 150 million stars, telling us where everything is going over time. It will be a mind bending accomplishment. Hipparchus would be proud.
With the most precise measurements, taken year after year, the motions of the stars can indeed be calculated. Although they’re not enough to see with the unaided eye, over thousands and tens of thousands of years, the positions of the stars change dramatically in the sky.
The familiar stars in the Big Dipper, for example, look how they do today. But if you go forward or backward in time, the positions of the stars look very different, and eventually completely unrecognizable.
When a star is moving sideways across the sky, astronomers call this “proper motion”. The speed a star moves is typically about 0.1 arc second per year. This is almost imperceptible, but over the course of 2000 years, for example, a typical star would have moved across the sky by about half a degree, or the width of the Moon in the sky.
The star with the fastest proper motion that we know of is Barnard’s star, zipping through the sky at 10.25 arcseconds a year. In that same 2000 year period, it would have moved 5.5 degrees, or about 11 times the width of your hand. Very fast.
When a star is moving toward or away from us, astronomers call that radial velocity. They measure this by calculating the doppler shift. The light from stars moving towards us is shifted towards the blue side of the spectrum, while stars moving away from us are red-shifted.
Between the proper motion and redshift, you can get a precise calculation for the exact path a star is moving in the sky.
We know, for example, that the dwarf star Hipparcos 85605 is moving rapidly towards us. It’s 16 light-years away right now, but in the next few hundred thousand years, it’s going to get as close as .13 light-years away, or about 8,200 times the distance from the Earth to the Sun. This won’t cause us any direct effect, but the gravitational interaction from the star could kick a bunch of comets out of the Oort cloud and send them down towards the inner Solar System.
The motions of the stars is fairly gentle, jostling through gravitational interactions as they orbit around the center of the Milky Way. But there are other, more catastrophic events that can make stars move much more quickly through space.
When a binary pair of stars gets too close to the supermassive black hole at the center of the Milky Way, one can be consumed by the black hole. The other now has the velocity, without the added mass of its companion. This gives it a high-velocity kick. About once every 100,000 years, a star is kicked right out of the Milky Way from the galactic center.
Another situation can happen where a smaller star is orbiting around a supermassive companion. Over time, the massive star bloats up as supergiant and then detonates as a supernova. Like a stone released from a sling, the smaller star is no longer held in place by gravity, and it hurtles out into space at incredible speeds.
Astronomers have detected these hypervelocity stars moving at 1.1 million kilometers per hour relative to the center of the Milky Way.
All of the methods of stellar motion that I talked about so far are natural. But can you imagine a future civilization that becomes so powerful it could move the stars themselves?
In 1987, the Russian astrophysicist Leonid Shkadov presented a technique that could move a star over vast lengths of time. By building a huge mirror and positioning it on one side of a star, the star itself could act like a thruster.
Photons from the star would reflect off the mirror, imparting momentum like a solar sail. The mirror itself would be massive enough that its gravity would attract the star, but the light pressure from the star would keep it from falling in. This would create a slow but steady pressure on the other side of the star, accelerating it in whatever direction the civilization wanted.
Over the course of a few billion years, a star could be relocated pretty much anywhere a civilization wanted within its host galaxy.
This would be a true Type III Civilization. A vast empire with such power and capability that they can rearrange the stars in their entire galaxy into a configuration that they find more useful. Maybe they arrange all the stars into a vast sphere, or some kind of geometric object, to minimize transit and communication times. Or maybe it makes more sense to push them all into a clean flat disk.
Amazingly, astronomers have actually gone looking for galaxies like this. In theory, a galaxy under control by a Type III Civilization should be obvious by the wavelength of light they give off. But so far, none have turned up. It’s all normal, natural galaxies as far as we can see in all directions.
For our short lifetimes, it appears as if the sky is frozen. The stars remain in their exact positions forever, but if you could speed up time, you’d see that everything is in motion, all the time, with stars moving back and forth, like airplanes across the sky. You just need to be patient to see it.
This star is X light-years away, that galaxy is X million light-years away. That beginning the Universe is X billion light-years away. But how do astronomers know?
I’m perpetually in a state where I’m talking about objects which are unimaginably far away. It’s pretty much impossible to imagine how huge some our Universe is. Our brains can comprehend the distances around us, sort of, especially when we’ve got a pile of tools to help. We can measure our height with a tape measure, or the distance along the ground using an odometer. We can get a feel for how far away 100 kilometers is because we can drive it in a pretty short period of time.
But space is really big, and for most of us, our brains can’t comprehend the full awesomeness of the cosmos, let alone measure it. So how do astronomers figure out how far away everything is? How do they know how far away planets, stars, galaxies, and even the edge of the observable Universe is? Assuming it’s all trickery? You’re bang on.
Astronomers have a bag of remarkably clever tricks and techniques to measure distance in the Universe. For them, different distances require a different methodologies. Up close, they use trigonometry, using differences in angles to puzzle out distances. They also use a variety of standard candles, those are bright objects that generate a consistent amount of light, so you can tell how far away they are. At the furthest distances, astronomers use expansion of space itself to detect distances.
Fortunately, each of these methods overlap. So you can use trigonometry to test out the closest standard candles. And you can use the most distant standard candles to verify the biggest tools. Around our Solar System, and in our neighborhood of the galaxy, astronomers use trigonometry to discover the distance to objects.
They measure the location of a star in the sky at one point of the year, and then measure again 6 months later when the Earth is on the opposite side of the Solar System. The star will have moved a tiny amount in the sky, known as parallax. Because we know the distance from one side of the Earth’s orbit to the other, we can calculate the angles, and compute the distance to the star.
I’m sure you can spot the flaw, this method falls apart when the distance is so great that the star doesn’t appear to move at all. Fortunately, astronomers shift to a different method, observing a standard candle known as a Cepheid variable. These Cepheids are special stars that dim and brighten in a known pattern. If you can measure how quickly a Cepheid pulses, you can calculate its true luminosity, and therefore its distance.
Cepheids let you measure distances to nearby galaxies. Out beyond a few dozen megaparsecs, you need another tool: supernovae. In a very special type of binary star system, one star dies and becomes a white dwarf, while the other star lives on. The white dwarf begins to feed material off the partner star until it hits exactly 1.4 times the mass of the Sun. At this point, it detonates as a Type 1A supernova, generating an explosion that can be seen halfway across the Universe. Because these stars always explode with exactly the same amount of material, we can detect how far away they are, and therefore their absolute brightness.
At the greatest scales, astronomers use the Hubble Constant. This is the discovery by Edwin Hubble that the Universe is expanding in all directions. The further you look, the faster galaxies are speeding away from us. By measuring the redshift of light from a galaxy, you can tell how fast it’s moving away from us, and thus its approximate distance. At the very end of this scale is the Cosmic Microwave Background Radiation, the edge of the observable Universe, and the limit of how far we can see.
Astronomers are always looking for new types of standard candles, and have discovered all kinds of clever ways to measure distance. They measure the clustering of galaxies, beams of microwave radiation from stars, and the surface of red giant stars – all in the hopes of verifying the cosmic distance ladder. Measuring distance has been one of the toughest problems for astronomers to crack and their solutions have been absolutely ingenious. Thanks to them, we can have a sense of scale for the cosmos around us.
What concept in astronomy do you have the hardest time holding in your brain? Tell us, in the comments below.
And if you like what you see, come check out our Patreon page and find out how you can get these videos early while helping us bring you more great content!
Fall will soon be at our doorstep. But before the leaves change colors and the smell of pumpkin fills our coffee shops, the Pleiades star cluster will mark the new season with its earlier presence in the night sky.
The delicate grouping of blue stars has been a prominent sight since antiquity. But in recent years, the cluster has also been the subject of an intense debate, marking a controversy that has troubled astronomers for more than a decade.
Now, a new measurement argues that the distance to the Pleiades star cluster measured by ESA’s Hipparcos satellite is decidedly wrong and that previous measurements from ground-based telescopes had it right all along.
The Pleiades star cluster is a perfect laboratory to study stellar evolution. Born from the same cloud of gas, all stars exhibit nearly identical ages and compositions, but vary in their mass. Accurate models, however, depend greatly on distance. So it’s critical that astronomers know the cluster’s distance precisely.
A well pinned down distance is also a perfect stepping stone in the cosmic distance ladder. In other words, accurate distances to the Pleiades will help produce accurate distances to the farthest galaxies.
But accurately measuring the vast distances in space is tricky. A star’s trigonometric parallax — its tiny apparent shift against background stars caused by our moving vantage point — tells its distance more truly than any other method.
Originally the consensus was that the Pleiades are about 435 light-years from Earth. However, ESA’s Hipparcos satellite, launched in 1989 to precisely measure the positions and distances of thousands of stars using parallax, produced a distance measurement of only about 392 light-years, with an error of less than 1%.
“That may not seem like a huge difference, but, in order to fit the physical characteristics of the Pleiades stars, it challenged our general understanding of how stars form and evolve,” said lead author Carl Melis, of the University of California, San Diego, in a press release. “To fit the Hipparcos distance measurement, some astronomers even suggested that some type of new and unknown physics had to be at work in such young stars.”
If the cluster really was 10% closer than everyone had thought, then the stars must be intrinsically dimmer than stellar models suggested. A debate ensued as to whether the spacecraft or the models were at fault.
To solve the discrepancy, Melis and his colleagues used a new technique known as very-long-baseline radio interferometry. By linking distant telescopes together, astronomers generate a virtual telescope, with a data-gathering surface as large as the distances between the telescopes.
The network included the Very Long Baseline Array (a system of 10 radio telescopes ranging from Hawaii to the Virgin Islands), the Green Bank Telescope in West Virginia, the William E. Gordon Telescope at the Arecibo Observatory in Puerto Rico, and the Effelsberg Radio Telescope in Germany.
“Using these telescopes working together, we had the equivalent of a telescope the size of the Earth,” said Amy Miouduszewski, of the National Radio Astronomy Observatory (NRAO). “That gave us the ability to make extremely accurate position measurements — the equivalent of measuring the thickness of a quarter in Los Angeles as seen from New York.”
After a year and a half of observations, the team determined a distance of 444.0 light-years to within 1% — matching the results from previous ground-based observations and not the Hipparcos satellite.
“The question now is what happened to Hipparcos?” Melis said.
The spacecraft measured the position of roughly 120,000 nearby stars and — in principle — calculated distances that were far more precise than possible with ground-based telescopes. If this result holds up, astronomers will grapple with why the Hipparcos observations misjudged the distances so badly.
ESA’s long-awaited Gaia observatory, which launched on Dec. 19, 2013, will use similar technology to measure the distances of about one billion stars. Although it’s now ready to begin its science mission, the mission team will have to take special care, utilizing the work of ground-based radio telescopes in order to ensure their measurements are accurate.
The findings have been published in the Aug. 29 issue of Science and is available online.
In astronomy we throw around the term “light-year” seemingly as fast as light itself travels. And yet actually measuring this distance is incredibly tricky. A star’s parallax — its tiny apparent shift once a year caused by our moving viewpoint on Earth — tells its distance more truly than any other method.
Accurate parallaxes of nearby stars form the base of the entire cosmic distance ladder out to the farthest galaxies. It’s a crucial science that’s about to take a giant leap forward. The European Space Agency’s long-awaited Gaia observatory — launched on Dec. 19, 2013 — is now ready to begin its science mission. Continue reading “GAIA is “Go” for Science After a few Minor Hiccups”
If you’re into astronomy, or just a fan of any science fiction franchise worth its salt, then chances are you’ve heard the term parsec thrown around. But what is a parsec exactly? Basically, it’s a unit of length used to measure the astronomically large distances between objects beyond our Solar System.
If you’re a semi-serious amateur astronomer, chances are you’ve heard of a variable pair of stars called SS Cygni. When you watch the system for long enough, you’re rewarded with a brightness outburst that then fades away and then returns, regularly, over and over again.
Turns out this bright pair is even closer to us than we imagined — 370 light-years away, to be precise.
Before we get into how this was discovered, a bit of background on what SS Cygni is. As the name of the system implies, it’s in the constellation of Cygnus (the Swan). The pair consists of a cooling white dwarf star that is locked in a 6.6-hour orbit with a red dwarf.
The white dwarf’s gravity, which is much stronger than that of the red dwarf, is bleeding material from its neighbor. This interaction causes outbursts — on average, about once every 50 days.
Previously, the Hubble Space Telescope put the distance to these stars much further away, at 520 light-years. But that caused some head-scratching among astronomers.
“That was a problem. At that distance, SS Cygni would have been the brightest dwarf nova in the sky, and should have had enough mass moving through its disk to remain stable without any outbursts,” stated James Miller-Jones, of the Curtin University node of the International Centre for Radio Astronomy Research in Perth, Australia.
Astronomers call SS Cygni a dwarf nova. When comparing it to similar systems, astronomers said the outbursts happen as matter changes its flow speed through the disc of material surrounding the white dwarf.
“At high rates of mass transfer from the red dwarf, the rotating disk remains stable, but when the rate is lower, the disk can become unstable and undergo an outburst,” stated the National Radio Astronomy Observatory. So what was happening?
To again look at the distance of the star, astronomers used two sets of radio telescopes, the Very Large Baseline Array and the European VLBI Network. Each set has a bunch of telescopes working together as an interferometer, allowing for precise measurements of star distances.
Scientists then took measurements at opposite ends of the Earth’s orbit, using the planet itself as a tool. By measuring the star’s distance at opposite sides of the orbit, we can calculate its parallax or apparent movement in the sky from the perspective of Earth. It’s an old astronomical tool used to pin down distances, and still works.
“This is one of the best-studied systems of its type, but according to our understanding of how these things work, it should not have been having outbursts. The new distance measurement brings it into line with the standard explanation,” stated Miller-Jones.
And where did Hubble go wrong? Here’s the theory:
“The radio observations were made against a background of objects far beyond our own Milky Way Galaxy, while the Hubble observations used stars within our galaxy as reference points,” NRAO stated. “The more-distant objects provide a better, more stable, reference.”
The results were published in Science on May 24.
Measuring distance doesn’t sound like a very challenging thing to do — just pick your standard unit of choice and corresponding tool calibrated to it, and see how the numbers add up. Use a meter stick, a tape measure, or perhaps take a drive, and you can get a fairly accurate answer. But in astronomy, where the distances are vast and there’s no way to take measurements in person, how do scientists know how far this is from that and what’s going where?
Luckily there are ways to figure such things out, and the methods that astronomers use are surprisingly familiar to things we experience every day.
[/caption]The video above is shared by the Royal Observatory Greenwich and shows how geometry, physics and things called “standard candles” (brilliant!) allow scientists to measure distances on cosmic scales.
Just in time for the upcoming transit of Venus, an event which also allows for some important measurements to be made of distances in our solar system, the video is part of a series of free presentations the Observatory is currently giving regarding our place in the Universe and how astronomers over the centuries have measured how oh-so-far it really is from here to there.
Video credits:
Design and direction: Richard Hogg
Animation: Robert Milne, Ross Philips, Kwok Fung Lam
Music and sound effects: George Demure
Narration and Astro-smarts: Dr. Olivia Johnson
Producer: Henry Holland