Our solar system has had a chaotic past. Earth and the other planets are now in stable orbits, but while they were forming they experienced drastic location shifts. Jupiter was likely much closer to the Sun than it is now, and its shift not only shifted other planets but also cleared the solar system of debris, tossing much of it to the Oort Cloud.
Continue reading “There Could Be Captured Planets in the Oort Cloud”The Oort Cloud Could Have More Rock Than Previously Believed
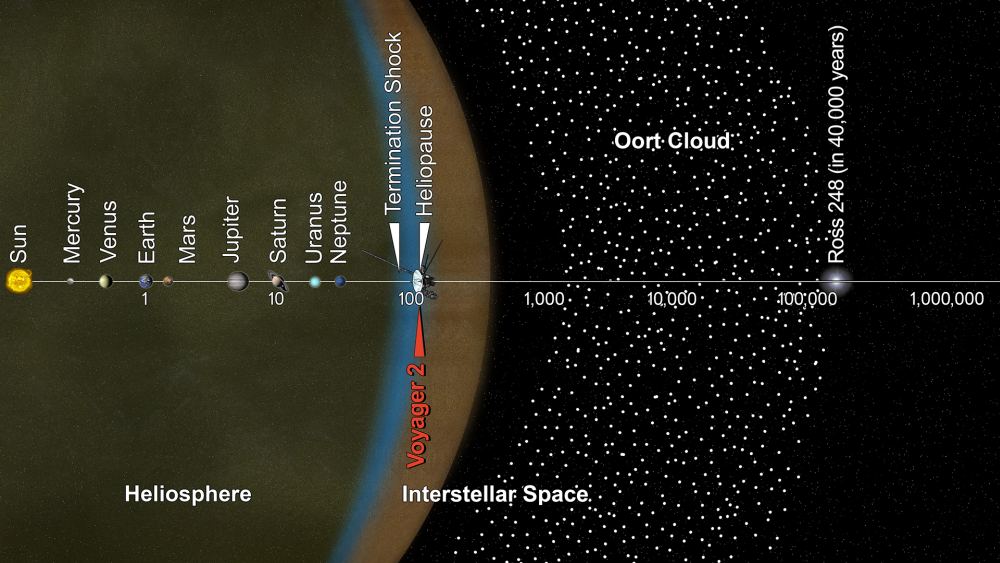
The Oort Cloud is a collection of icy objects in the furthest reaches of the Solar System. It contains the most distant objects in the Solar System, and instead of orbiting on a plane like the planets or forming a ring like the Kuiper Belt, it’s a vast spherical cloud centred on the Sun. It’s where comets originate, and beyond it is interstellar space.
At least that’s what scientists think; nobody’s ever seen it.
Continue reading “The Oort Cloud Could Have More Rock Than Previously Believed”Researchers Simulate the Formation of the Oort Cloud
There is a cloud of debris surrounding our solar system. It’s known as the Oort cloud, and it is the source of most of the comets in our solar system. It was first proposed by Jan Oort, as a way to explain why there were so many long-period comets, and why they can appear from almost any direction. It’s estimated that there are about 100 billion small icy bodies in the Oort cloud, spread throughout a sphere about 50,000 AU from the Sun. Through our studies of comets we’ve learned a great deal about the Oort cloud, but we still don’t fully understand how it came to be.
Continue reading “Researchers Simulate the Formation of the Oort Cloud”In 1.3 Million Years, a Star Will Come Within 24 Light-Days of the Sun

Within the Milky Way, there are an estimated 200 to 400 billion stars, all of which orbit around the center of our galaxy in a coordinated cosmic dance. As they orbit, stars in the galactic disk (where our Sun is located) periodically shuffle about and get closer to one another. At times, this can have a drastic effect on the star that experience a close encounter, disrupting their systems and causing planets to be ejected.
Knowing when stars will make a close encounter with our Solar System, and how it might shake-up objects within it, is therefore a concern to astronomers. Using data collected by the Gaia Observatory, two researchers with the Russian Academy of Sciences (RAS) determined that a handful of stars will be making close passes by our Solar System in the future, one of which will stray pretty close!
Continue reading “In 1.3 Million Years, a Star Will Come Within 24 Light-Days of the Sun”The Sun Might Have Once Had a Binary Companion Star
For some time now, astronomers have known that the majority of systems in our galaxy consist of binary pairs rather than individual stars. What’s more, in recent decades, research has revealed that stars like our Sun are actually born in clusters within solar nebulas. This has led to efforts in recent years to locate G-type (yellow dwarf) stars in our galaxy that could be the Sun’s long-lost “solar siblings.”
And now, a new study by Harvard astronomers Amir Siraj and Prof. Abraham Loeb has shown that the Sun may once have once had a very similar binary companion that got kicked out of our Solar System. If confirmed, the implications of this could be groundbreaking, especially where theories on how the Oort Cloud formed and whether or not our system captured a massive object (Planet Nine) in the past.
Continue reading “The Sun Might Have Once Had a Binary Companion Star”Oort Clouds Around Other Stars Should be Visible in the Cosmic Microwave Background
For decades, scientists have theorized that beyond the edge of the Solar System, at a distance of up to 50,000 AU (0.79 ly) from the Sun, there lies a massive cloud of icy planetesimals known as the Oort Cloud. Named in honor of Dutch astronomer Jan Oort, this cloud is believed to be where long-term comets originate from. However, to date, no direct evidence has been provided to confirm the Oort Cloud’s existence.
This is due to the fact that the Oort Cloud is very difficult to observe, being rather far from the Sun and dispersed over a very large region of space. However, in a recent study, a team of astrophysicists from the University of Pennsylvania proposed a radical idea. Using maps of the Cosmic Microwave Background (CMB) created by the Planck mission and other telescopes, they believe that Oort Clouds around other stars can be detected.
The study – “Probing Oort clouds around Milky Way stars with CMB surveys“, which recently appeared online – was led by Eric J Baxter, a postdoctoral researcher from the Department of Physics and Astronomy at the University of Pennsylvania. He was joined by Pennsylvania professors Cullen H. Blake and Bhuvnesh Jain (Baxter’s primary mentor).
To recap, the Oort Cloud is a hypothetical region of space that is thought to extend from between 2,000 and 5,000 AU (0.03 and 0.08 ly) to as far as 50,000 AU (0.79 ly) from the Sun – though some estimates indicate it could reach as far as 100,000 to 200,000 AU (1.58 and 3.16 ly). Like the Kuiper Belt and the Scattered Disc, the Oort Cloud is a reservoir of trans-Neptunian objects, though it is over a thousands times more distant from our Sun as these other two.
This cloud is believed to have originated from a population of small, icy bodies within 50 AU of the Sun that were present when the Solar System was still young. Over time, it is theorized that orbital perturbations caused by the giant planets caused those objects that had highly-stable orbits to form the Kuiper Belt along the ecliptic plane, while those that had more eccentric and distant orbits formed the Oort Cloud.
According to Baxter and his colleagues, because the existence of the Oort Cloud played an important role in the formation of the Solar System, it is therefore logical to assume that other star systems have their own Oort Clouds – which they refer to as exo-Oort Clouds (EXOCs). As Dr. Baxter explained to Universe Today via email:
“One of the proposed mechanisms for the formation of the Oort cloud around our sun is that some of the objects in the protoplanetary disk of our solar system were ejected into very large, elliptical orbits by interactions with the giant planets. The orbits of these objects were then affected by nearby stars and galactic tides, causing them to depart from orbits restricted to the plane of the solar system, and to form the now-spherical Oort cloud. You could imagine that a similar process could occur around another star with giant planets, and we know that there are many stars out there that do have giant planets.”
As Baxter and his colleagues indicated in their study, detecting EXOCs is difficult, largely for the same reasons for why there is no direct evidence for the Solar System’s own Oort Cloud. For one, there is not a lot of material in the cloud, with estimates ranging from a few to twenty times the mass of the Earth. Second, these objects are very far away from our Sun, which means they do not reflect much light or have strong thermal emissions.
For this reason, Baxter and his team recommended using maps of the sky at the millimeter and submillimeter wavelengths to search for signs of Oort Clouds around other stars. Such maps already exist, thanks to missions like the Planck telescope which have mapped the Cosmic Microwave Background (CMB). As Baxter indicated:
“In our paper, we use maps of the sky at 545 GHz and 857 GHz that were generated from observations by the Planck satellite. Planck was pretty much designed *only* to map the CMB; the fact that we can use this telescope to study exo-Oort clouds and potentially processes connected to planet formation is pretty surprising!”
This is a rather revolutionary idea, as the detection of EXOCs was not part of the intended purpose of the Planck mission. By mapping the CMB, which is “relic radiation” left over from the Big Bang, astronomers have sought to learn more about how the Universe has evolved since the the early Universe – circa. 378,000 years after the Big Bang. However, their study does build on previous work led by Alan Stern (the principal investigator of the New Horizons mission).
In 1991, along with John Stocke (of the University of Colorado, Boulder) and Paul Weissmann (from NASA’s Jet Propulsion Laboratory), Stern conducted a study titled “An IRAS search for extra-solar Oort clouds“. In this study, they suggested using data from the Infrared Astronomical Satellite (IRAS) for the purpose of searching for EXOCs. However, whereas this study focused on certain wavelengths and 17 star systems, Baxter and his team relied on data for tens of thousands of systems and at a wider range of wavelengths.
Other current and future telescopes which Baxter and his team believe could be useful in this respect include the South Pole Telescope, located at the Amundsen–Scott South Pole Station in Antarctica; the Atacama Cosmology Telescope and the Simons Observatory in Chile; the Balloon-borne Large Aperture Submillimeter Telescope (BLAST) in Antarctica; the Green Bank Telescope in West Virgina, and others.
“Furthermore, the Gaia satellite has recently mapped out very accurately the positions and distances of stars in our galaxy,” Baxter added. “This makes choosing targets for exo-Oort cloud searches relatively straightforward. We used a combination of Gaia and Planck data in our analysis.”
To test their theory, Baxter and is team constructed a series of models for the thermal emission of exo-Oort clouds. “These models suggested that detecting exo-Oort clouds around nearby stars (or at least putting limits on their properties) was feasible given existing telescopes and observations,” he said. “In particular, the models suggested that data from the Planck satellite could potentially come close to detecting an exo-Oort cloud like our own around a nearby star.”
In addition, Baxter and his team also detected a hint of a signal around some of the stars that they considered in their study – specifically in the Vega and Formalhaut systems. Using this data, they were able to place constraints on the possible existence of EXOCs at a distance of 10,000 to 100,000 AUs from these stars, which roughly coincides with the distance between our Sun and the Oort Cloud.
However, additional surveys will be needed before the existence any of EXOCs can be confirmed. These surveys will likely involve the James Webb Space Telescope, which is scheduled to launch in 2021. In the meantime, this study has some rather significant implications for astronomers, and not just because it involves the use of existing CMB maps for extra-solar studies. As Baxter put it:
“Just detecting an exo-Oort cloud would be really interesting, since as I mentioned above, we don’t have any direct evidence for the existence of our own Oort cloud. If you did get a detection of an exo-Oort cloud, it could in principle provide insights into processes connected to planet formation and the evolution of protoplanetary disks. For instance, imagine that we only detected exo-Oort clouds around stars that have giant planets. That would provide pretty convincing evidence that the formation of an Oort cloud is connected to giant planets, as suggested by popular theories of the formation of our own Oort cloud.”
Further Reading: arXiv
Now We Know When Stars Will Be Passing Through the Oort Cloud
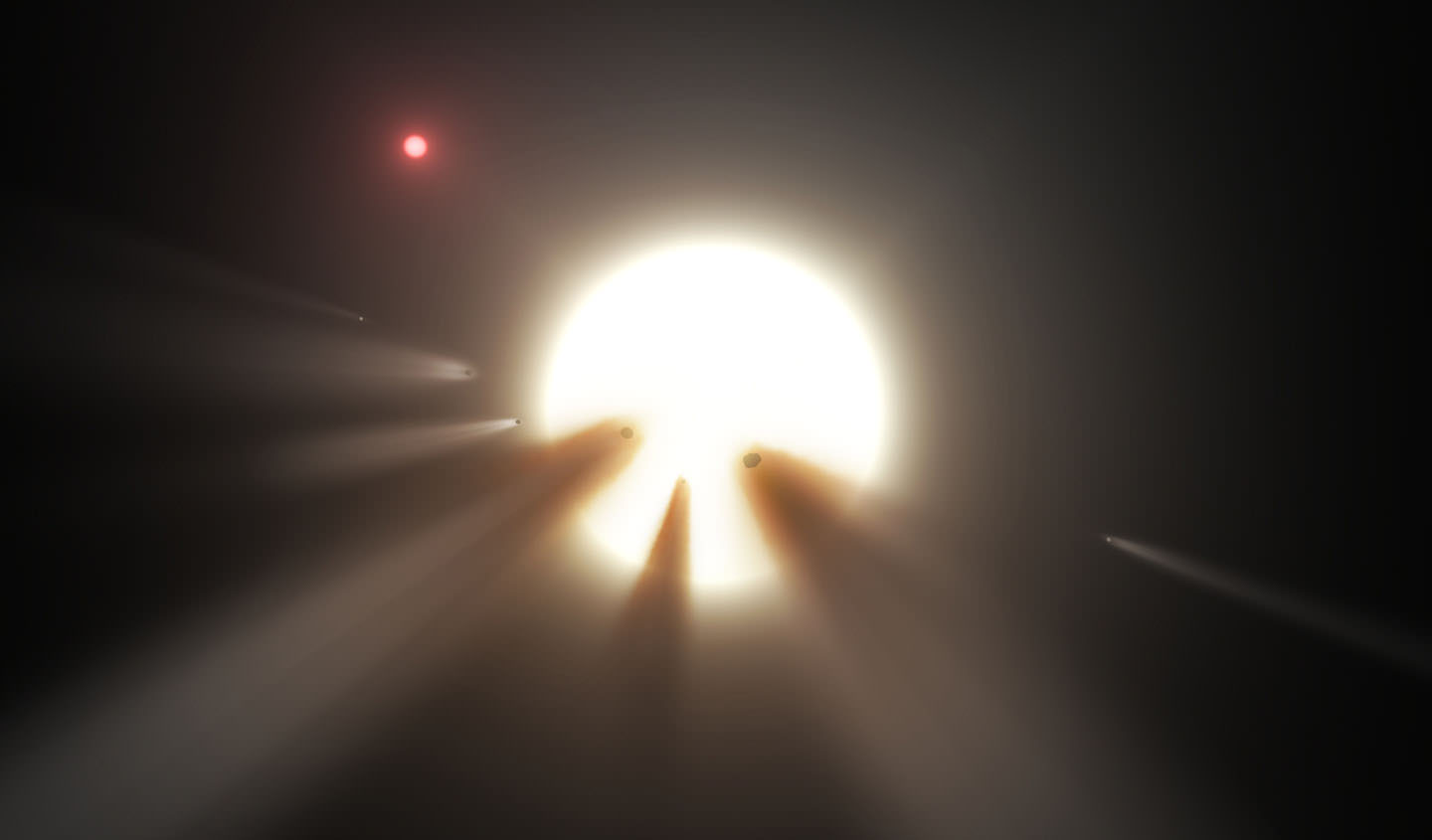
To our Solar System, “close-encounters” with other stars happen regularly – the last occurring some 70,000 years ago and the next likely to take place 240,000 to 470,000 years from now. While this might sound like a “few and far between” kind of thing, it is quite regular in cosmological terms. Understanding when these encounters will happen is also important since they are known to cause disturbances in the Oort Cloud, sending comets towards Earth.
Thanks to a new study by Coryn Bailer-Jones, a researcher from the Max Planck Institute for Astronomy, astronomers now have refined estimates on when the next close-encounters will be happening. After consulting data from the ESA’s Gaia spacecraft, he concluded that over the course of the next 5 million years, that the Solar System can expect 16 close encounters, and one particularly close one!
For the sake of the study – which recently appeared in the journal Astronomy & Astrophysics under the title “The Completeness-Corrected Rate of Stellar Encounters with the Sun From the First Gaia Data Release” – Dr. Bailer Jones used Gaia data to track the movements of more than 300,000 stars in our galaxy to see if they would ever pass close enough to the Solar System to cause a disturbance.
As noted, these types of disturbances have happened many times throughout the history of the Solar System. In order to dislodge icy objects from their orbit in the Oort Cloud – which extends out to about 15 trillion km (100,000 AU) from our Sun – and send them hurling into the inner Solar System, it is estimated that a star would need to pass within 60 trillion km (37 trillion mi; 400,000 AU) of our Sun.
While these close encounters pose no real risk to our Solar System, they have been known to increase comet activity. As Dr. Bailer-Jones explained to Universe Today via email:
“Their potential influence is to shake up the Oort cloud of comets surrounding our Sun, which could result in some being pushed into the inner solar system where is chance they could impact with the Earth. But the long-term probability of one such comet hitting the Earth is probably lower than the probability the Earth is hit by a near-Earth asteroid. So they don’t pose much more danger.”
One of the goals of the Gaia mission, which launched back in 2013, was to collect precise data on stellar positions and motions over the course of its five-year mission. After 14 months in space, the first catalogue was released, which contained information on more than a billion stars. This catalogue also contained the distances and motions across the sky of over two million stars.
By combining this new data with existing information, Dr. Bailer-Jones was able to calculate the motions of some 300,000 stars relative to the Sun over a five million year period. As he explained:
“I traced the orbits of stars observed by Gaia (in the so-called TGAS catalogue) backwards and forwards in time, to see when and how close they would come to the Sun. I then computed the so-called ‘completeness function’ of TGAS to find out what fraction of encounters would have been missed by the survey: TGAS doesn’t see fainter stars (and the very brightest stars are also omitted at present, for technical reasons), but using a simple model of the Galaxy I can estimate how many stars it is missing. Combining this with the actual number of encounters found, I could estimate the total rate of stellar encounters (i.e. including the ones not actually seen). This is necessarily a rather rough estimate, as it involves a number of assumptions, not least the model for what is not seen.”
From this, he was able to come up with a general estimate of the rate of stellar encounters over the past 5 million years, and for the next 5 million. He determined that the overall rate is about 550 stars per million years coming within 150 trillion km, and about 20 coming closer than 30 trillion km. This works out to about one potential close encounter every 50,000 years or so.
Dr. Bailor-Jones also determined that of the 300,000 stars he observed, 97 of them would pass within 150 trillion km (93 trillion mi; 1 million AU) of our Solar System, while 16 would come within 60 trillion km. While this would be close enough to disturb the Oort Cloud, only one star would get particularly close. That star is Gliese 710, a K-type yellow dwarf located about 63 light years from Earth which is about half the size of our Sun.
According to Dr. Bailer-Jones’ study, this star will pass by our Solar System in 1.3 million years, and at a distance of just 2.3 trillion km (1.4 trillion mi; 16 ,000AU). This will place it well within the Oort Cloud, and will likely turn many icy planetesimals into long-period comets that could head towards Earth. What’s more, Gliese 710 has a relatively slow velocity compared to other stars in our galaxy.
Whereas the average relative velocity of stars is estimated to be around 100.000 km/h (62,000 mph) at their closest approach, Gliese 710 will will have a speed of 50,000 km/h (31,000 mph). As a result, the star will have plenty of time to exert its gravitational influence on the Oort Cloud, which could potentially send many, many comets towards Earth and the inner Solar System.
Over the past few decades, this star has been well-documented by astronomers, and they were already pretty certain that it would experience a close encounter with our Solar System in the future. However, previous calculations indicated that it would pass within 3.1 to 13.6 trillion km (1.9 to 8.45 trillion mi; 20,722 to 90,910 AU) from our star system – and with a 90% certainty. Thanks to this most recent study, these estimates have been refined to 1.5–3.2 trillion km, with 2.3 trillion km being the most likely.
Again, while it might sound like these passes are on too large of a timescale to be of concern, in terms of the astronomical history, its a regular occurrence. And while not every close encounter is guaranteed to send comets hurling our way, understanding when and how these encounters have happened is intrinsic to understanding the history and evolution of our Solar System.
Understanding when a close encounters might happen next is also vital. Assuming we are still around when another takes place, knowing when it is likely to happen could allow us to prepare for the worst – i.e. if a comets is set on a collision course with Earth! Failing that, humanity could use this information to prepare a scientific mission to study the comets that are sent our way.
The second release of Gaia data is scheduled for next April, and will contain information on an estimated 1 billion stars. That’s 20 times as many stars as the first catalogue, and about 1% the total number of stars within the Milky Way Galaxy. The second catalog will also include information on much more distant stars, will which allow for reconstructions of up to 25 million years into the past and future.
As Dr. Bailer-Jones indicated, the release of Gaia data has helped astronomers considerably. “[I]t greatly improves on what we had before, in both number of stars and precision,” he said. “But this is really just a taster of what will come in the second data release in April 2018, when we will provide parallaxes and proper motions for around one billion stars (500 times as many as in the first data release).”
With every new release, estimates on the movements of the galaxy’s stars (and the potential for close encounters) will be refined further. It will also help us to chart when major comet activity took place within the Solar System, and how this might have played a role in the evolution of the planets and life itself.
Further Reading: ESA
Colonizing the Outer Solar System
Okay, so this article is Colonizing the Outer Solar System, and is actually part 2 of our team up with Fraser Cain of Universe Today, who looked at colonizing the inner solar system. You might want jump over there now and watch that part first, if you are coming in from having seen part 1, welcome, it is great having you here.
Without further ado let us get started. There is no official demarcation between the inner and outer solar system but for today we will be beginning the outer solar system at the Asteroid Belt.
The Asteroid Belt is always of interest to us for colonization. We have talked about mining them before if you want the details on that but for today I’ll just remind everyone that there are very rich in metals, including precious metals like gold and platinum, and that provides all the motivation we need to colonize them. We have a lot of places to cover so we won’t repeat the details on that today.
You cannot terraform asteroids the way you could Venus or Mars so that you could walk around on them like Earth, but in every respect they have a lot going for them as a candidate. They’ve got plenty for rock and metal for construction, they have lots of the basic organic elements, and they even have some water. They also get a decent amount of sunlight, less than Mars let alone Earth, but still enough for use as a power source and to grow plants.
But they don’t have much gravity, which – pardon the pun – has its ups and downs. There just isn’t much mass in the Belt. The entire thing has only a small fraction of the mass of our moon, and over half of that is in the four biggest asteroids, essentially dwarf planets in their own right. The remainder is scattered over millions of asteroids. Even the biggest, Ceres, is only about 1% of 1% of Earth’s mass, has a surface gravity of 3% Earth-normal, and an escape velocity low enough most model rockets could get into orbit. And again, it is the biggest, most you could get away from by jumping hard and if you dropped an object on one it might take a few minutes to land.
You can still terraform one though, by definition too. The gentleman who coined the term, science fiction author Jack Williamson, who also coined the term genetic engineering, used it for a smaller asteroid just a few kilometers across, so any definition of terraforming has to include tiny asteroids too.
Of course in that story it’s like a small planet because they had artificial gravity, we don’t, if we want to fake gravity without having mass we need to spin stuff around. So if we want to terraform an asteroid we need to hollow it out and fill it with air and spin it around.
Of course you do not actually hollow out the asteroid and spin it, asteroids are loose balls of gravel and most would fly apart given any noticeable spin. Instead you would hollow it out and set a cylinder spinning inside it. Sort of like how a good thermos has an outside container and inside one with a layer of vacuum in between, we would spin the inner cylinder.
You wouldn’t have to work hard to hollow out an asteroid either, most aren’t big enough to have sufficient gravity and pressure to crush an empty beer can even at their center. So you can pull matter out from them very easily and shore up the sides with very thin metal walls or even ice. Or just have your cylinder set inside a second non-spinning outer skin or superstructure, like your washer or dryer.
You can then conduct your mining from the inside, shielded from space. You could ever pressurize that hollowed out area if your spinning living area was inside its own superstructure. No gravity, but warmth and air, and you could get away with just a little spin without tearing it apart, maybe enough for plants to grow to normally.
It should be noted that you can potentially colonize even the gas giants themselves, even though our focus today is mostly on their moons. That requires a lot more effort and technology then the sorts of colonies we are discussing today, Fraser and I decided to keep things near-future and fairly low tech, though he actually did an article on colonizing Jupiter itself last year that was my main source material back before got to talking and decided to do a video together.
Hydrogen is plentiful on Jupiter itself and floating refineries or ships that fly down to scoop it up might be quite useful, but again today we are more interested in its moons. The biggest problem with colonizing the moons of Jupiter is all the radiation the planet gives off.
Europa is best known as a place where the surface is covered with ice but beneath it is thought to be a vast subsurface ocean. It is the sixth largest moon coming right behind our own at number five and is one of the original four moons Galileo discovered back in 1610, almost two centuries before we even discovered Uranus, so it has always been a source of interest. However as we have discovered more planets and moons we have come to believe quite a few of them might also have subsurface oceans too.
Now what is neat about them is that water, liquid water, always leaves the door open to the possibility of life already existing there. We still know so little about how life originally evolved and what conditions permit that to occur that we cannot rule out places like Europa already having their own plants and animals swimming around under that ice.
They probably do not and obviously we wouldn’t want to colonize them, beyond research bases, if they did, but if they do not they become excellent places to colonize. You could have submarine cities in such places floating around in the sea or those buried in the surface ice layer, well shielded from radiation and debris. The water also geysers up to the surface in some places so you can start off near those, you don’t have to drill down through kilometers of ice on day one.
Water, and hydrogen, are also quite uncommon in the inner solar system so having access to a place like Europa where the escape velocity is only about a fifth of our own is quite handy for export. Now as we move on to talk about moons a lot it is important to note that when I say something has a fifth of the escape velocity of Earth that doesn’t mean it is fives time easier to get off of. Energy rises with the square of velocity so if you need to go five times faster you need to spend 5-squared or 25 times more energy, and even more if that place has tons of air creating friction and drag, atmospheres are hard to claw your way up through though they make landing easier too. But even ignoring air friction you can move 25 liters of water off of Europa for every liter you could export from Earth and even it is a very high in gravity compared to most moons and comets. Plus we probably don’t want to export lots of water, or anything else, off of Earth anyway.
We should start by noting two things. First, the Asteroid Belt is not the only place you find asteroids, Jupiter’s Trojan Asteroids are nearly as numerous, and every planet, including Earth, has an equivalent to Jupiter’s Trojan Asteroids at its own Lagrange Points with the Sun. Though just as Jupiter dwarfs all the other planets so to does its collection of Lagrangian objects. They can quite big too, the largest 624 Hektor, is 400 km across, and has a size and shape similar to Pennsylvania.
And as these asteroids are at stable Lagrange Points, they orbit with Jupiter but always ahead and behind it, making transit to and from Jupiter much easier and making good waypoints.
Before we go out any further in the solar system we should probably address how you get the energy to stay alive. Mars is already quite cold compared to Earth, and the Asteroids and Jupiter even more so, but with thick insulation and some mirrors to bounce light in you can do fairly decently. Indeed, sunlight out by Jupiter is already down to just 4% of what Earth gets, meaning at Jovian distances it is about 50 W/m²
That might not sound like much but it is actually almost a third of what average illumination is on Earth, when you factor in atmospheric reflection, cloudy days, nighttime, and higher, colder latitudes. It is also a good deal brighter than the inside of most well-lit buildings, and is enough for decently robust photosynthesis to grow food. Especially with supplemental light from mirrors or LED growth lamps.
But once you get out to Saturn and further that becomes increasingly impractical and a serious issue, because while food growth does not show up on your electric bill it is what we use virtually all our energy for. Closer in to the sun we can use solar panels for power and we do not need any power to grow food. As we get further out we cannot use solar and we need to heat or cold habitats and supply lighting for food, so we need a lot more power even as our main source dries up.
So what are our options? Well the first is simple, build bigger mirrors. A mirror can be quite large and paper thin after all. Alternatively we can build those mirrors far away, closer to the sun, and and either focus them on the place we want illuminated or send an energy beam, microwaves perhaps or lasers, out to the destination to supply energy.
We also have the option of using fission, if we can find enough Uranium or Thorium. There is not a lot of either in the solar system, in the area of about one part per billion, but that does amount to hundreds of trillions of tons, and it should only take a few thousand tons a year to supply Earth’s entire electric grid. So we would be looking at millions of years worth of energy supply.
Of course fusion is even better, particularly since hydrogen becomes much more abundant as you get further from the Sun. We do not have fusion yet, but it is a technology we can plan around probably having inside our lifetimes, and while uranium and thorium might be counted in parts per billion, hydrogen is more plentiful than every other element combines, especially once you get far from the Sun and Inner Solar System.
So it is much better power source, an effectively unlimited one except on time scales of billions and trillion of years. Still, if we do not have it, we still have other options. Bigger mirrors, beaming energy outwards from closer to the Sun, and classic fission of Uranium and Thorium. Access to fusion is not absolutely necessary but if you have it you can unlock the outer solar system because you have your energy supply, a cheap and abundant fuel supply, and much faster and cheaper spaceships.
Of course hydrogen, plain old vanilla hydrogen with one proton, like the sun uses for fusion, is harder to fuse than deuterium and may be a lot longer developing, we also have fusion using Helium-3 which has some advantages over hydrogen, so that is worth keeping in mind as well as we proceed outward.
Okay, let’s move on to Saturn, and again our focus is on its moons more than the planet itself. The biggest of those an the most interesting for colonization is Titan.
Titan is aptly named, this titanic moon contains more mass than than all of Saturn’s sixty or so other moons and by an entire order of magnitude at that. It is massive enough to hold an atmosphere, and one where the surface pressure is 45% higher than here on Earth. Even though Titan is much smaller than Earth, its atmosphere is about 20% more massive than our own. It’s almost all nitrogen too, even more than our own atmosphere, so while you would need a breather mask to supply oxygen and it is also super-cold, so you’d need a thick insulated suit, it doesn’t have to be a pressure suit like it would on Mars or almost anyplace else.
There’s no oxygen in the atmosphere, what little isn’t nitrogen is mostly methane and hydrogen, but there is plenty of oxygen in the ice on Titan which is quite abundant. So it has everything we need for life except energy and gravity. At 14% of earth normal it is probably too low for people to comfortably and safely adapt to, but we’ve already discussed ways of dealing with that. It is low enough that you could probably flap your arms and fly, if you had wing attached.
It needs some source of energy though, and we discussed that. Obviously if you’ve got fusion you have all the hydrogen you need, but Titan is one of those places we would probably want to colonize early on if we could, it is something you need a lot of to terraform other places, and is also rich in a lot of the others things we want. So we often think of it as a low-tech colony since it is one we would want early on.
In an scenario like that it is very easy to imagine a lot of local transit between Titan and its smaller neighboring moons, which are more rocky and might be easier to dig fissile materials like Uranium and Thorium out of. You might have a dozen or so small outposts on neighboring moons mining fissile materials and other metals and a big central hub on Titan they delivered that too which also exported Nitrogen to other colonies in the solar system.
Moving back and forth between moons is pretty easy, especially since things landing on Titan can aerobrake quite easily, whereas Titan itself has a pretty strong gravity well and thick atmosphere to climb out of but is a good candidate for a space elevator, since it requires nothing more sophisticated than a Lunar Elevator on our own moon and has an abundant supply of the materials needed to make Zylon for instance, a material strong enough to make an elevator there and which we can mass manufacture right now.
Titan might be the largest and most useful of Saturn’s moons, but again it isn’t the only one and not all of the other are just rocks for mining. At last count it has over sixty and many of them quite large. One of those, Enceladus, Saturn’s sixth largest moon, is a lot like Jupiter’s Moon Europa, in that we believe it has a large and thick subsurface ocean. So just like Europa it is an interesting candidate for Colonization. So Titan might be the hub for Saturn but it wouldn’t be the only significant place to colonize.
While Saturn is best known for its amazing rings, they tend to be overlooked in colonization. Now those rings are almost all ice and in total mass about a quarter as much as Enceladus, which again is Saturn’s Sixth largest moon, which is itself not even a thousandth of the Mass of Titan.
In spite of that the rings are not a bad place to set up shop. Being mostly water, they are abundant in hydrogen for fusion fuel and have little mass individually makes them as easy to approach or leave as an asteroid. Just big icebergs in space really, and there are many moonlets in the rings that can be as large as half a kilometer across. So you can burrow down inside one for protection from radiation and impacts and possibly mine smaller ones for their ice to be brought to places where water is not abundant.
In total those rings, which are all frozen water, only mass about 2% of Earth’s oceans, and about as much as the entire Antarctic sheet. So it is a lot of fresh water that is very easy to access and move elsewhere, and ice mines in the rings of Saturn might be quite useful and make good homes. Living inside an iceball might not sound appealing but it is better than it sounds like and we will discuss that more when we reach the Kupier Belt.
But first we still have two more planets to look at, Uranus and Neptune.
Uranus, and Neptune, are sometimes known as Ice Giants instead of Gas Giants because it has a lot more water. It also has more ammonia and methane and all three get called ices in this context because they make up most of the solid matter when you get this far out in the solar system.
While Jupiter is over a thousand times the mass of Earth, Uranus weighs in at about 15 times the Earth and has only about double the escape velocity of Earth itself, the least of any of the gas giants, and it’s strange rotation, and its strange tilt contributes to it having much less wind than other giants. Additionally the gravity is just a little less than Earth’s in the atmosphere so we have the option for floating habitats again, though it would be a lot more like a submarine than a hot air balloon.
Like Venus, Uranus has very long days, at least in terms of places receiving continual sunlight, the poles get 42 years of perpetual sunlight then 42 of darkness. Sunlight being a relative term, the light is quite minimal especially inside the atmosphere. The low wind in many places makes it a good spot for gas extraction, such as Helium-3, and it’s a good planet to try to scoop gas from or even have permanent installations.
Now Uranus has a large collection of moons as well, useful and colonizable like the other moons we have looked at, but otherwise unremarkable beyond being named for characters from Shakespeare, rather than the more common mythological names. None have atmospheres though there is a possibility Oberon or Titania might have subsurface oceans.
Neptune makes for a brief entry, it is very similar to Uranus except it has the characteristically high winds of gas giants that Uranus’s skewed poles mitigate, meaning it has no advantages over Uranus and the disadvantages of high wind speeds everywhere and being even further from the Sun. It too has moons and one of them, Triton, is thought to have subsurface oceans as well. Triton also presumably has a good amount of nitrogen inside it since it often erupts geysers of nitrogen from its surface.
Triton is one of the largest moons in the solar system, coming in seventh just after our Moon, number 5, and Europa at number 6. Meaning that were it not a moon it would probably qualify as a Dwarf Planet and it is often thought Pluto might be an escaped moon Neptune. So Triton might be one that didn’t escape, or didn’t avoid getting captured. In fact there are an awful lot of bodies in this general size range and composition wandering about in the outer regions of our solar system as we get out into the Kuiper Belt.
The Kuiper Belt is one of those things that has a claim on the somewhat arbitrary and hazy boundary marking the edge of the Solar System. It extends from out past Neptune to beyond Pluto and contains a good deal more mass than the asteroid Belt. It is where a lot of our comets come from and while there is plenty of rocks out there they tend to be covered in ice. In other words it is like our asteroid belt only there’s more of it and the one thing the belt is not very abundant in, water and hydrogen in general, is quite abundant out there. So if you have a power source life fusion they can be easily terraformed and are just as attractive as a source of minerals as the various asteroids and moons closer in.
We mentioned the idea of living inside hollowed out asteroids earlier and you can use the same trick for comets. Indeed you could shape them to be much bigger if you like, since they would be hollow and ice isn’t hard to move and shape especially in zero gravity. Same trick as before, you place a spinning cylinder inside it. Not all the objects entirely ice and indeed your average comet is more a frozen ball of mud then ice with rocky cores. We think a lot of near Earth Asteroids are just leftover comets. So they are probably pretty good homes if you have fusion, lots of fuel and raw materials for both life and construction.
This is probably your cheapest interstellar spacecraft too, in terms of effort anyway. People often talk about re-directing comets to Mars to bring it air and water, but you can just as easily re-direct it out of the solar system entirely. Comets tend to have highly eccentric orbits, so if you capture one when it is near the Sun you can accelerate it then, actually benefiting from the Oberth Effect, and drive it out of the solar system into deep space. If you have a fusion power source to live inside one then you also have an interstellar spaceship drive, so you just carve yourself a small colony inside the comet and head out into deep space.
You’ve got supplies that will last you many centuries at least, even if it were home to tens of thousand of people, and while we think of smaller asteroids and comets as tiny, that’s just in comparison to planets. These things tend to be the size of mountain so there is plenty of living space and a kilometer of dirty ice between you and space makes a great shield against even the kinds of radiation and collisions you can experience at relativistic speeds.
Now the Oort Cloud is much like the Kupier Belt but begins even further out and extends out probably an entire light year or more. We don’t have a firm idea of its exact dimensions or mass, but the current notion is that it has at least several Earth’s worth of mass, mostly in various icy bodies. These will be quite numerous, estimates usually assumes at least trillion icy bodies a kilometer across or bigger, and even more smaller ones. However the volume of space is so large that those kilometer wide bodies might each be a around a billion kilometers distant from neighbors, or about a light hour. So it is spread out quite thinly, and even the inner edge is about 10 light days away.
That means that from a practical standpoint there is no source of power out there, the sun is simply too diffuse for even massive collections of mirrors and solar panels to be of use. It also means light-speed messages home or to neighbors are quite delayed. So in terms of communication it is a lot more like pre-modern times in sparsely settled lands where talking with your nearest neighbors might require an hour long walk over to their farm, and any news from the big cities might take months to percolate out to you.
There’s probably uranium and thorium out there to be found, maybe a decent amount of it, so fission as a power source is not ruled out. If you have fusion instead though each of these kilometer wide icy bodies is like a giant tank of gasoline, and as with the Kupier Belt, ice makes a nice shield against impacts and radiation.
And while there might be trillions of kilometer wide chunks of ice out there, and many more smaller bodies, you would have quite a few larger ones too. There are almost certainly tons of planets in the Pluto size-range out these, and maybe even larger ones. Even after the Oort cloud you would still have a lot of these deep space rogue planets which could bridge the gap to another solar system’s Oort Cloud. So if you have fusion you have no shortage of energy, and could colonize trillions of these bodies. There probably is a decent amount of rock and metal out there too, but that could be your major import/export option shipping home ice and shipping out metals.
That’s the edge of the Solar System so that’s the end of this article. If you haven’t already read the other half, colonizing the inner Solar System, head on over now.
Does Our Galaxy Have a Habitable Zone?
I’ve got to say, you are one of the luckiest people I’ve ever met.
For starters, you are the descendant of an incomprehensible number of lifeforms who were successful, and survived long enough to find a partner, procreate, and have an offspring. Billions of years, and you are the result of an unbroken chain of success, surviving through global catastrophe after catastrophe. Nice going.
Not only that, but your lineage happened to be born on a planet, which was in just the right location around just the right kind of star. Not too hot, not too cold, just the right temperature where liquid water, and whatever else was necessary for life to get going. Again, I like your lucky streak.
In fact, you happened to be born into a Universe that has the right physical constants, like the force of gravity or the binding force of atoms, so that stars, planets and even the chemistry of life could happen at all.
But there’s another lottery you won, and you probably didn’t even know about it. You happened to be born on an unassuming, mostly harmless planet orbiting a G-type main sequence star in the habitable zone of the Milky Way.
Wait a second, even galaxies have habitable zones? Yep, and you’re in it right now.
The Milky Way is a big place, measuring up to 180,000 light years across. It contains 100 to 400 billion stars spread across this enormous volume.
We’re located about 27,000 light years away from the center of the Milky Way, and tens of thousands of light-years away from the outer rim.
The Milky Way has some really uninhabitable zones. Down near the center of the galaxy, the density of stars is much greater. And these stars are blasting out a combined radiation that would make it much more unlikely for life to evolve.
Radiation is bad for life. But it gets worse. There’s a huge cloud of comets around the Sun known as the Oort Cloud. Some of the greatest catastrophes in history happened when these comets were kicked into a collision course with the Earth by a passing star. Closer to the galactic core, these disruptions would happen much more often.
There’s another dangerous place you don’t want to be: the galaxy’s spiral arms. These are regions of increased density in the galaxy, where star formation is much more common. And newly forming stars blast out dangerous radiation.
Fortunately, we’re far away from the spiral arms, and we orbit the center of the Milky Way in a nice circular orbit, which means we don’t cross these spiral arms very often.
We stay nice and far away from the dangerous parts of the Milky Way, however, we’re still close enough to the action that our Solar System gathered the elements we needed for life.
The first stars in the Universe only had hydrogen, helium and a few other trace elements left over from the Big Bang. But when the largest stars detonated as supernovae, they seeded the surrounding regions with heavier elements like oxygen, carbon, even iron and gold.
Our solar nebula was seeded with the heavy elements from many generations of stars, giving us all the raw materials to help set evolution in motion.
If the Solar System was further out, we probably wouldn’t have gotten enough of those heavier elements. So, thanks multiple generations of dead stars.
According to astrobiologists the galactic habitable zone probably starts just outside the galactic bulge – about 13,000 light-years from the center, and ends about halfway out in the disk, 33,000 light-years from the center.
Remember, we’re 27,000 light-years from the center, so just inside that outer edge. Phew.
Of course, not all astronomers believe in this Rare Earth hypothesis. In fact, just as we’re finding life on Earth wherever we find water, they believe that life is more robust and resilient. It could still survive and even thrive with more radiation, and less heavier elements.
Furthermore, we’re learning that solar systems might be able to migrate a significant distance from where they formed. Stars that started closer in where there were plenty of heavier elements might have drifted outward to the safer, calmer galactic suburbs, giving life a better chance at getting a foothold.
As always, we’ll need more data, more research to get an answer to this question.
Just when you thought you were already lucky, it turns out you were super duper extra lucky. Right Universe, right lineage, right solar system, right location in the Milky Way. You already won the greatest lottery in existence.
Planet 9 Search Turning Up Wealth Of New Objects

In 2014, Scott Sheppard of the Carnegie Institution for Science and Chadwick Trujillo of Northern Arizona University proposed an interesting idea. Noting the similarities in the orbits of distant Trans-Neptunian Objects (TNOs), they postulated that a massive object was likely influencing them. This was followed in 2016 by Konstantin Batygin and Michael E. Brown of Caltech suggesting that an undiscovered planet was the culprit.
Since that time, the hunt has been on for the infamous “Planet 9” in our Solar System. And while no direct evidence has been produced, astronomers believe they are getting closer to discerning its location. In a paper that was recently accepted by The Astronomical Journal, Sheppard and Trujillo present their latest discoveries, which they claim are further constraining the location of Planet 9.
For the sake of their study, Sheppard and Trujillo relied on information obtained by the Dark Energy Camera on the Victor Blanco 4-meter telescope in Chile and the Japanese Hyper Suprime-Camera on the 8-meter Subaru Telescope in Hawaii. With the help of David Tholen from the University of Hawaii, they have been conducting the largest deep-sky survey for objects beyond Neptune and the Kuiper Belt.
This survey is intended to find more objects that show the same clustering in their orbits, thus offering greater evidence that a massive planet exists in the outer Solar System. As Sheppard explained in a recent Carnegie press release:
“Objects found far beyond Neptune hold the key to unlocking our Solar System’s origins and evolution. Though we believe there are thousands of these small objects, we haven’t found very many of them yet, because they are so far away. The smaller objects can lead us to the much bigger planet we think exists out there. The more we discover, the better we will be able to understand what is going on in the outer Solar System.”
Their most recent discovery was a small collection of more extreme objects who’s peculiar orbits differ from the extreme and inner Oort cloud objects, in terms of both their eccentricities and semi-major axes. As with discoveries made using other instruments, these appear to indicate the presence of something massive effecting their orbits.
All of these objects have been submitted to the International Astronomical Union’s (IAU) Minor Planet Center for designation. They include 2014 SR349, an extreme TNO that has similar orbital characteristics as the previously-discovered extreme bodies that led Sheppard and Trujillo to infer the existence of a massive object in the region.
Another is 2014 FE72, an object who’s orbit is so extreme that it reaches about 3000 AUs from the Sun in a massively-elongated ellipse – something which can only be explained by the influence of a strong gravitational force beyond our Solar System. And in addition to being the first object observed at such a large distance, it is also the first distant Oort Cloud object found to orbit entirely beyond Neptune.
And then there’s 2013 FT28, which is similar but also different from the other extreme objects. For instance, 2013 FT28 shows similar clustering in terms of its semi-major axis, eccentricity, inclination, and argument of perihelion angle, but is different when it comes to its longitude of perihelion. This would seem to indicates that this particular clustering trend is less strong among the extreme TNOs.
Beyond the work of Sheppard and Trujillo, nearly 10 percent of the sky has now been explored by astronomers. Relying on the most advanced telescopes, they have revealed that there are several never-before-seen objects that orbit the Sun at extreme distances.
And as more distant objects with unexplained orbital parameters emerge, their interactions seem to fit with the idea of a massive distant planet that could pay a key role in the mechanics of the outer Solar System. However, as Sheppard has indicated, there really isn’t enough evidence yet to draw any conclusions.
“Right now we are dealing with very low-number statistics, so we don’t really understand what is happening in the outer Solar System,” he said. “Greater numbers of extreme trans-Neptunian objects must be found to fully determine the structure of our outer Solar System.”
Alas, we don’t yet know if Planet 9 is out there, and it will probably be many more years before confirmation can be made. But by looking to the visible objects that present a possible sign of its path, we are slowly getting closer to it. With all the news in exoplanet hunting of late, it is interesting to see that we can still go hunting in our own backyard!
Further Reading: The Astrophysical Journal Letters