The gravitational wave background was first detected in 2016. It was announced following the release of the first data set from the European Pulsar Timing Array. A second set of data has just been released and, joined by the Indian Pulsar Timing Array, both studies confirm the existence of the background. The latest theory seems to suggest that we’re seeing the combined signal of supermassive black hole mergers.
Continue reading “More Evidence for the Gravitational Wave Background of the Universe”Astronomers Will Get Gravitational Wave Alerts Within 30 Seconds
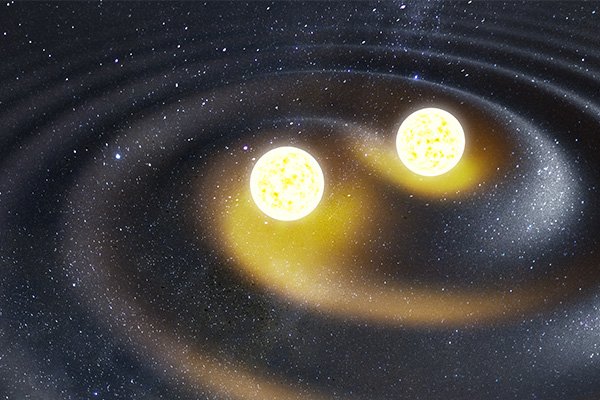
Any event in the cosmos generates gravitational waves, the bigger the event, the more disturbance. Events where black holes and neutron stars collide can send out waves detectable here on Earth. It is possible that there can be an event in visible light when neutron stars collide so to take advantage of every opportunity an early warning is essential. The teams at LIGO-Virgo-KAGRA observatories are working on an alert system that will alert astronomers within 30 seconds fo a gravity wave event. If warning is early enough it may be possible to identify the source and watch the after glow.
Continue reading “Astronomers Will Get Gravitational Wave Alerts Within 30 Seconds”Astronomers Figure Out How to use Gravitational Lensing to Measure the Mass of White Dwarfs
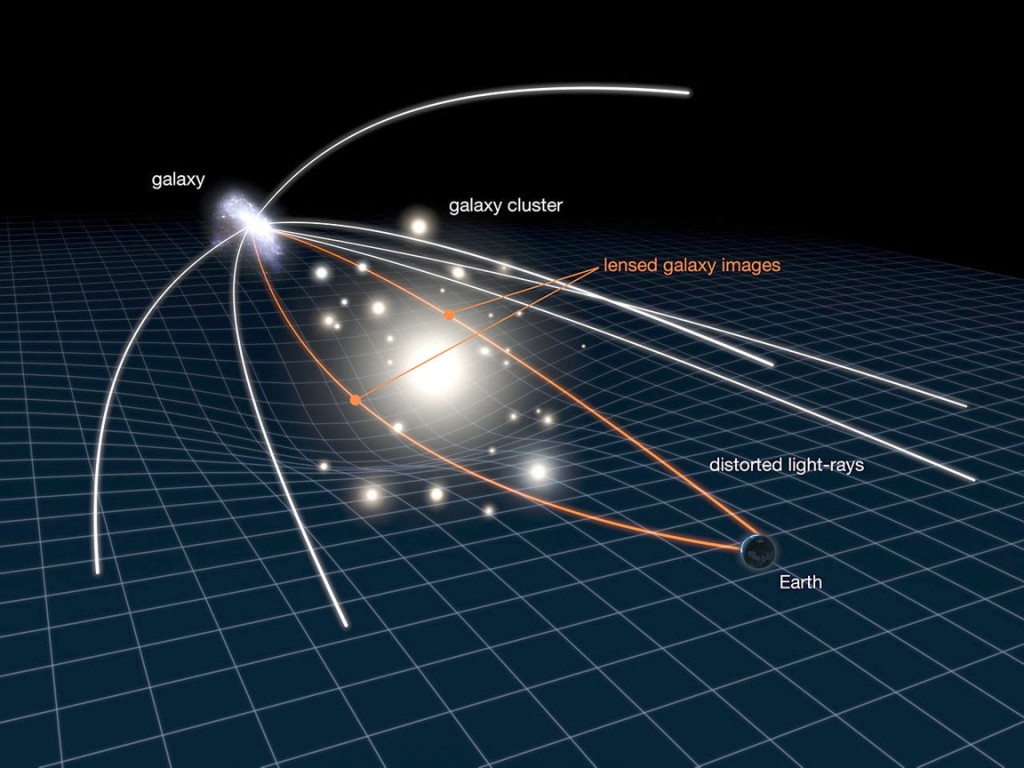
For the sake of studying the most distant objects in the Universe, astronomers often rely on a technique known as Gravitational Lensing. Based on the principles of Einstein’s Theory of General Relativity, this technique involves relying on a large distribution of matter (such as a galaxy cluster or star) to magnify the light coming from a distant object, thereby making it appear brighter and larger.
However, in recent years, astronomers have found other uses for this technique as well. For instance, a team of scientists from the Harvard-Smithsonian Center for Astrophysics (CfA) recently determined that Gravitational Lensing could also be used to determine the mass of white dwarf stars. This discovery could lead to a new era in astronomy where the mass of fainter objects can be determined.
The study which details their findings, titled “Predicting gravitational lensing by stellar remnants” appeared in the Monthly Noticed of the Royal Astronomical Society. The study was led by Alexander J. Harding of the CfA and included Rosanne Di Stefano, and Claire Baker (also from the CfA), as well as members from the University of Southampton, Georgia State University, the University of Nigeria, and Cornell University.
To put it simply, determining the mass of an astronomical object is one the greatest challenges for astronomers. Until now, the most successful method relied on binary systems because the orbital parameters of these systems depend on the masses of the two objects. Unfortunately, objects that are at the end states of stellar evolution – like black holes, neutron stars or white dwarfs – are often too faint or isolated to be detectable.
This is unfortunate, since these objects are responsible for a lot of dramatic astronomical events. These include the accretion of material, the emission of energetic radiation, gravitational waves, gamma-ray bursts, or supernovae. Many of these events are still a mystery to astronomers or the study of them is still in its infancy – i.e. gravitational waves. As they state in their study:
“Gravitational lensing provides an alternative approach to mass measurement. It has the advantage of only relying on the light from a background source, and can therefore be employed even for dark lenses. In fact, since light from the lens can interfere with the detection of lensing effects, compact objects are ideal lenses.”
As they go on to state, of the 18,000 lensing events that have been detected to date, roughly 10 to 15% are believed to have been caused by compact objects. However, scientists are unable to tell which of the detected events were due to compact lenses. For the sake of their study then, the team sought to circumvent this problem by identifying local compact objects and predicting when they might produce a lensing event so they could be studied.
“By focusing on pre-selected compact objects in the near vicinity of the Sun, we ensure that the lensing event will be caused by a white dwarf, neutron star, or black hole,” they state. “Furthermore, the distance and proper motion of the lens can be accurately measured prior to the event, or else afterwards. Armed with this information, the lensing light curve allows one to accurately measure the mass of the lens.”
In the end, the team determined that lensing events could be predicted from thousands of local objects. These include 250 neutron stars, 5 black holes, and roughly 35,000 white dwarfs. Neutron stars and black holes present a challenge since the known populations are too small and their proper motions and/or distances are not generally known.
But in the case of white dwarfs, the authors anticipate that they will provide for many lensing opportunities in the future. Based on the general motions of the white dwarfs across the sky, they obtained a statistical estimate that about 30-50 lensing events will take place per decade that could be spotted by the Hubble Space Telescope, the ESA’s Gaia mission, or NASA’s James Webb Space Telescope (JWST). As they state in their conclusions:
“We find that the detection of lensing events due to white dwarfs can certainly be observed during the next decade by both Gaia and HST. Photometric events will occur, but to detect them will require observations of the positions of hundreds to thousands of far-flung white dwarfs. As we learn the positions, distances to, and proper motions of larger numbers of white dwarfs through the completion of surveys such as Gaia and through ongoing and new wide-field surveys, the situation will continue to improve.”
The future of astronomy does indeed seem bright. Between improvements in technology, methodology, and the deployment of next-generation space and ground-based telescopes, there is no shortage of opportunities to see and learn more.
Are Aliens Communicating with Neutrino Beams?
It is no easy thing to search for signs of intelligent life beyond our Solar System. In addition to the incredible distances involved and the fact that we really only have indirect methods at our disposal, there is also the small problem of not knowing exactly what to look for. If intelligent life does exist beyond our Solar System, would they even communicate as we do, using radio transmitters and similar forms of technology?
Such has been the preoccupation of groups like the Search for Extra Terrestrial Intelligence (SETI) Institute and, more recently, organizations like Messaging Extraterrestrial Intelligence (METI) International. A non-profit dedicated to communicating with extra-terrestrial intelligence (ETI), the organization recently suggested that looking for neutrinos and other exotic particles could help us find signals as well.
First, some clarification should be made as to what SETI and METI are all about it and what sets them apart. The term METI was coined by Russian scientist Alexander Zaitsev, who sought to draw a distinction between SETI and METI. As he explained in a 2006 paper on the subject:
“The science known as SETI deals with searching for messages from aliens. METI science deals with the creation of messages to aliens. Thus, SETI and METI proponents have quite different perspectives. SETI scientists are in a position to address only the local question “does Active SETI make sense?” In other words, would it be reasonable, for SETI success, to transmit with the object of attracting ETI’s attention? In contrast to Active SETI, METI pursues not a local and lucrative impulse, but a more global and unselfish one – to overcome the Great Silence in the Universe, bringing to our extraterrestrial neighbors the long-expected annunciation ‘You are not alone!'”
In short, METI looks for ways in which we might be able to contact aliens instead of waiting to hear from them. However, this does not mean that organizations like METI International are without ideas on how me might better listen to our (potential) alien neighbors. After all, communication goes beyond mere messages, and also requires that a medium exist with which to convey the message.
Such is the recommendation put forth by Dr. Morris Jones, a space analyst and writer who serves on the METI advisory council. In a recent article published on METI International’s website, he addressed the two main challenges when it comes to looking for ETI. On the one hand, you have the need for multiple methodologies to increase the odds of finding something. But as he indicates, there’s also the problem of knowing what to look for:
“We are not really sure of how extraterrestrials would communicate with us. Would they use radio waves, lasers, or something more exotic? Perhaps the universe is awash in extraterrestrial signals that we cannot even receive. SETI and METI practitioners spend a lot of time wondering how a message would be encoded in terms of language and content. It’s also important to consider the medium of transmission.”
In the past, says Jones, SETI searches were based on radio astronomy because that was the only practical means of doing so. Since then, efforts have expanded to include optical telescopes and the search for laser signals. This is due to the fact that in the past few decades, human beings have developed the technology to use laser for the sake of communications.
In a 2016 SETI paper, Dr. Philip Lubin of the University of California, Santa Barbara, explained how the development of directed-energy propulsion could help us search for evidence of aliens. As one of the scientific minds behind Breakthrough Starshot – a laser-driven lightsail that would be fast enough to make the trip to Alpha Centauri in just 20 years – he believes it’s a safe bet that ETI could be using similar technology to travel or communicate.
In addition, Dr. Avi Loeb from the Harvard-Smithsonian Center for Astrophysics (also one of the minds behind Starshot) has also suggested that fast-radio bursts (FRBs) could be evidence of alien activity. FRBs have been a subject of fascination to scientists since they were first detected in 2007 (the “Lorimer Burst“), and could also be a sign of alien communications or a means of propulsion.
Another means involves searching for artefacts – i.e. looking for evidence of physical infrastructure in other star systems. Case in point, since 2015, astronomers have been seeking to determine what is responsible for the periodic dimming of KIC 8462852 (aka. Tabby’s Star). Whereas most studies have sought to explain this in terms of natural causes, others have suggested it could be evidence of an alien megastructure.
To this array of search methods, Dr. Jones offers a few other possibilities. One way is to look for neutrinos, a type of subatomic particle that is produced by the decay of radioactive elements and interacts with matter very weakly. This allows them to pass through solid matter and also makes them very difficult to detect. Neutrinos are produced in large quantities by our Sun and astronomical sources, but they can also be produced artificially by nuclear reactors.
These, claims Jones, could be used for the sake of communications. The only problem is that looking for them would require some specialized equipment. Currently, all means of detecting neutrinos involve expensive facilities that have to be built either underground or in extremely isolated locations to ensure that they are not subject to any kind of electromagnetic interference.
These include the Super-Kamiokande facility, the world’s largest neutrino detector which is located under Mt. Ikeno in Japan. There’s also the IceCube Neutrino Observatory, located at the Amundsen–Scott South Pole Station in Antarctica and operated by the University of Wisconsin–Madison; and the Sudbury Neutrino Observatory, located in a former mine complex near Sudbury, Ontario, and operated by SNOLAB.
Another possibility is searching for evidence of communications that rely on gravitational waves. Predicted by Einstein’s Theory of General Relativity, the first detection of these mysterious waves was first made in February 2016. And in the coming years and decades, it is expected that gravitational wave observatories will be established so the presence of these “ripples” in spacetime can be visualized.
However, compared to neutrinos, Jones admits that this seems like a long shot. “It’s hard to conceive with our current grasp of physics,” he writes. “They are extremely difficult to generate at a detectable level. You would need abilities similar to those of superheroes, and be able to smash neutron stars and black holes together at will. There are probably easier ways to get a message across the stars.”
Beyond these, there is the even more exotic possibility of “Zeta Rays”, which Dr. Jones is not prepared to rule out. Basically, “Zeta Rays” is a term used by physicists to describe physics that go beyond the Standard Model. As scientists are currently looking for evidence of new particles with the Large Hadron Collider and other particle accelerators, it stands to reason that anything they discover will be the added to the SETI and METI search manifest.
But could such physics entail new forms of communication? Hard to say, but definitely worth considering. After all, the physics that power our current technology certainly existed before we did. Or as Jones put it:,
“Is it possible to transmit with something better than we already have? Until we know a lot more physics, we just won’t know. Humanity in the twenty-first century could be like an isolated tribe in the Amazon jungle a century ago, unaware that the air around them was filled with radio signals. SETI uses the science and technology provided to us by other disciplines. Thus, we must wait until physics itself makes some more major breakthroughs. Only then can we consider such exotic methods of searching. We think a lot about the message. But we should also think about the medium.”
Other projects that are dedicated to METI include Breakthrough Listen, a ten-year initiative launched by Breakthrough Initiatives to conduct the largest survey to date for extraterrestrial communications – encompassing the 1,000,000 closest stars and 100 closest galaxies. Back in April of 2017, the scientists behind this project shared their analysis of the first year of Listen data. No definitive results have been announced yet, but they are just getting started!
Ever since Drake proposed his famous equation, human beings have eagerly sought to find evidence of extra-terrestrial intelligence. Unfortunately, all of our efforts have been haunted by Fermi’s equally-famous paradox! But of course, as space exploration goes, we’ve really only begun to scratch the surface of our Universe. And the only way we can ever expect to find evidence of intelligent life out there is to keep looking.
And with greater knowledge and increasingly sophisticated methods at our disposal, we can be sure that if intelligent life is out there somewhere, we will find it eventually. One can always hope, right? And be sure to check out this video of Dr. Jones 2014 presentation at the SETI Institute, titled “A Journalistic Perspective on SETI-Related Message Composition“:
Further Reading: METI
How We Know Gravity is Not (Just) a Force

When we think of gravity, we typically think of it as a force between masses. When you step on a scale, for example, the number on the scale represents the pull of the Earth’s gravity on your mass, giving you weight. It is easy to imagine the gravitational force of the Sun holding the planets in their orbits, or the gravitational pull of a black hole. Forces are easy to understand as pushes and pulls.
But we now understand that gravity as a force is only part of a more complex phenomenon described the theory of general relativity. While general relativity is an elegant theory, it’s a radical departure from the idea of gravity as a force. As Carl Sagan once said, “Extraordinary claims require extraordinary evidence,” and Einstein’s theory is a very extraordinary claim. But it turns out there are several extraordinary experiments that confirm the curvature of space and time.
The key to general relativity lies in the fact that everything in a gravitational field falls at the same rate. Stand on the Moon and drop a hammer and a feather, and they will hit the surface at the same time. The same is true for any object regardless of its mass or physical makeup, and this is known as the equivalence principle.
Since everything falls in the same way regardless of its mass, it means that without some external point of reference, a free-floating observer far from gravitational sources and a free-falling observer in the gravitational field of a massive body each have the same experience. For example, astronauts in the space station look as if they are floating without gravity. Actually, the gravitational pull of the Earth on the space station is nearly as strong as it is at the surface. The difference is that the space station (and everything in it) is falling. The space station is in orbit, which means it is literally falling around the Earth.
This equivalence between floating and falling is what Einstein used to develop his theory. In general relativity, gravity is not a force between masses. Instead gravity is an effect of the warping of space and time in the presence of mass. Without a force acting upon it, an object will move in a straight line. If you draw a line on a sheet of paper, and then twist or bend the paper, the line will no longer appear straight. In the same way, the straight path of an object is bent when space and time is bent. This explains why all objects fall at the same rate. The gravity warps spacetime in a particular way, so the straight paths of all objects are bent in the same way near the Earth.
So what kind of experiment could possibly prove that gravity is warped spacetime? One stems from the fact that light can be deflected by a nearby mass. It is often argued that since light has no mass, it shouldn’t be deflected by the gravitational force of a body. This isn’t quite correct. Since light has energy, and by special relativity mass and energy are equivalent, Newton’s gravitational theory predicts that light would be deflected slightly by a nearby mass. The difference is that general relativity predicts it will be deflected twice as much.
The effect was first observed by Arthur Eddington in 1919. Eddington traveled to the island of Principe off the coast of West Africa to photograph a total eclipse. He had taken photos of the same region of the sky sometime earlier. By comparing the eclipse photos and the earlier photos of the same sky, Eddington was able to show the apparent position of stars shifted when the Sun was near. The amount of deflection agreed with Einstein, and not Newton. Since then we’ve seen a similar effect where the light of distant quasars and galaxies are deflected by closer masses. It is often referred to as gravitational lensing, and it has been used to measure the masses of galaxies, and even see the effects of dark matter.
Another piece of evidence is known as the time-delay experiment. The mass of the Sun warps space near it, therefore light passing near the Sun is doesn’t travel in a perfectly straight line. Instead it travels along a slightly curved path that is a bit longer. This means light from a planet on the other side of the solar system from Earth reaches us a tiny bit later than we would otherwise expect. The first measurement of this time delay was in the late 1960s by Irwin Shapiro. Radio signals were bounced off Venus from Earth when the two planets were almost on opposite sides of the sun. The measured delay of the signals’ round trip was about 200 microseconds, just as predicted by general relativity. This effect is now known as the Shapiro time delay, and it means the average speed of light (as determined by the travel time) is slightly slower than the (always constant) instantaneous speed of light.
A third effect is gravitational waves. If stars warp space around them, then the motion of stars in a binary system should create ripples in spacetime, similar to the way swirling your finger in water can create ripples on the water’s surface. As the gravity waves radiate away from the stars, they take away some of the energy from the binary system. This means that the two stars gradually move closer together, an effect known as inspiralling. As the two stars inspiral, their orbital period gets shorter because their orbits are getting smaller.
For regular binary stars this effect is so small that we can’t observe it. However in 1974 two astronomers (Hulse and Taylor) discovered an interesting pulsar. Pulsars are rapidly rotating neutron stars that happen to radiate radio pulses in our direction. The pulse rate of pulsars are typically very, very regular. Hulse and Taylor noticed that this particular pulsar’s rate would speed up slightly then slow down slightly at a regular rate. They showed that this variation was due to the motion of the pulsar as it orbited a star. They were able to determine the orbital motion of the pulsar very precisely, calculating its orbital period to within a fraction of a second. As they observed their pulsar over the years, they noticed its orbital period was gradually getting shorter. The pulsar is inspiralling due to the radiation of gravity waves, just as predicted.
Finally there is an effect known as frame dragging. We have seen this effect near Earth itself. Because the Earth is rotating, it not only curves spacetime by its mass, it twists spacetime around it due to its rotation. This twisting of spacetime is known as frame dragging. The effect is not very big near the Earth, but it can be measured through the Lense-Thirring effect. Basically you put a spherical gyroscope in orbit, and see if its axis of rotation changes. If there is no frame dragging, then the orientation of the gyroscope shouldn’t change. If there is frame dragging, then the spiral twist of space and time will cause the gyroscope to precess, and its orientation will slowly change over time.
We’ve actually done this experiment with a satellite known as Gravity Probe B, and you can see the results in the figure here. As you can see, they agree very well.
Each of these experiments show that gravity is not simply a force between masses. Gravity is instead an effect of space and time. Gravity is built into the very shape of the universe.
Think on that the next time you step onto a scale.
Weird Gravity Waves Pulse From a Tropical Cyclone
Last Monday, May 13, the Suomi NPP satellite captured a fascinating image of Tropical Cyclone Mahasen as it moved northeast over the Bay of Bengal. The clouds of the storm itself weren’t optically visible in the darkness of a nearly new Moon, but lightning flashes within it were… as well as the eerie ripples of atmospheric gravity waves spreading outwards from its center.
According to the Space Physics Research Group at the University of California, Berkeley:
Gravity waves are the oscillations of air parcels by the lifting force of bouyancy and the restoring force of gravity. These waves propagate vertically as well as horizontally, and actively transport energy and momentum from the troposphere to the middle and upper atmosphere. Gravity waves are caused by a variety of sources, including the passage of wind across terrestrial landforms, interaction at the velocity shear of the polar jet stream and radiation incident from space. They are found to affect atmospheric tides in the middle atmosphere and terrestrial weather in the lower atmosphere. (Source)
Atmospheric gravity waves aren’t to be confused with gravitational waves in space, which are created by very dense, massive objects (like white dwarf stars or black holes) orbiting each other closely.
When the image was captured, Tropical Cyclone Mahasen was moving north through the Indian Ocean along a track that placed landfall along the Bangladesh coast. As it moved off the coast of India Suomi’s VIIRS Day-Night Band was able to resolve lightning flashes towards the center of the storm, along with mesopheric gravity waves emanating outwards like ripples in a pond.
Such gravity waves are of particular interest to air traffic controllers so assist in identifying areas of turbulence.
Since the moon was in a new phase, the lights and other surface features of India and Sri Lanka are clearly visible although the clouds of Mahasen are not — a tradeoff that occurs as the amount of moonlight cycles throughout the month.
Over the course of the next few days Mahasen weakened into a deep depression, making landfall as a tropical storm on Bangladesh on May 16. In preparation for the storm large-scale evacuations were recommended for parts of Myanmar; however, this resulted in the overcrowding of boats and several vessels capsized. (Source: eosnap.com)
NASA launched the National Polar-orbiting Operational Environmental Satellite System Preparatory Project (or NPP) on October 28, 2011 from Vandenberg Air Force Base. On Jan. 24, NPP was renamed Suomi National Polar-orbiting Partnership, or Suomi NPP, in honor of the late Verner E. Suomi. It’s the first satellite specifically designed to collect data to improve short-term weather forecasts and increase understanding of long-term climate change.
Suomi NPP orbits Earth about 14 times a day, observing nearly the entire surface of the planet.
Main image source: NASA Goddard Space Flight Center
Extreme Telescopes: Unique Observatories Around the World
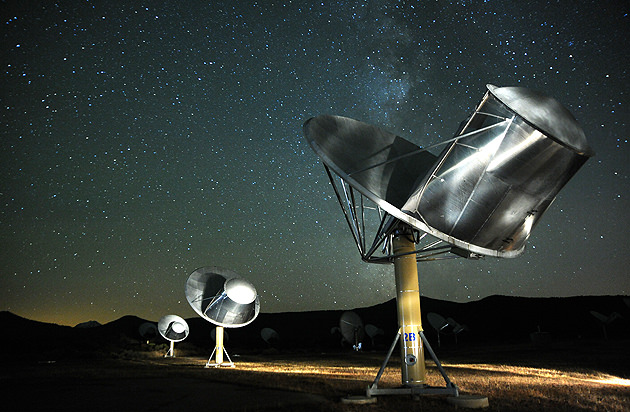
In 1888, astronomer Simon Newcomb uttered now infamous words, stating that “We are probably nearing the limit of all we can know about astronomy.” This was an age just prior to identifying faint nebulae as separate galaxies, Einstein’s theory of special and general relativity, and an era when a hypothetical substance called the aether was said to permeate the cosmos.
Newcomb would scarcely recognize astronomy today. Modern observatories span the electromagnetic spectrum and are unlocking the secrets of a universe both weird and wonderful. Modern day astronomers rarely peer through an eyepiece, were it even possible to do so with such bizarre instruments. What follows are some of the most unique professional ground-based observatories in operation today that are pushing back our understanding of the universe we inhabit.
VERITAS: Based at the Fred Lawrence Whipple Observatory in southern Arizona, the Very Energetic Radiation Imaging Telescope Array System (VERITAS) is an observatory designed to observe high energy gamma-rays. Its array consists of four 12-metre aperture reflectors each comprised of 350 mirror scintillators. Each VERITAS array has a 3.5° degree field of view and the array has been fully operational since 2007. VERITAS has been used to study active galactic nuclei, gamma-ray bursts, and the Crab Nebula pulsar.
IceCube: Not the rapper, IceCube is a neutrino detector in based at the Amundsen-Scott South Pole Station in Antarctica. IceCube watches for neutrino interactions by use of thousands of photomultipliers suspended up to 2.45 kilometres down into the Antarctic ice sheet. With a total of 86 detector strings completed in 2011, IceCube is currently the world’s largest neutrino observatory and is part of the worldwide Supernova Early Warning System. IceCube will also complement WMAP and Planck data and can actually “see” the shadowing effect of the Moon blocking cosmic ray muons.
Liquid Mirror Telescopes: One of the more bizarre optical designs out there in the world of astronomy, liquid mirror telescopes employ a large rotating dish of mercury to form a parabolic mirror. The design is cost effective but does have the slight drawback of having to aim directly at the zenith while a swath of sky passes over head. NASA employed a 3-metre liquid mirror telescope as part of its Orbital Debris observatory based near Cloudcroft, New Mexico from 1995-2002. The largest one in the world (and the 18th largest optical telescope overall) is the 6-metre Large Zenith Telescope in the University of British Columbia’s Malcolm Knapp Research Forest.
LIGO: Designed to detect incoming gravity waves caused by pulsar-black hole mergers, the Laser Interferometer Gravitational-Wave Observatory (LIGO) is comprised of a pair of facilities with one based in Hanford, Washington and another in Livingston, Louisiana. Each detector is consists of a pair of 2 kilometre Fabry-Pérot arms and measures a laser beam shot through them with ultra-high precision. Two geographically separate interferometers are needed to isolate out terrestrial interference as well as give a direction of an incoming gravity wave on the celestial sphere. To date, no gravity waves have been detected by LIGO, but said detection is expected to open up a whole new field of astronomy.
The Very Long Baseline Array: A series of 10 radio telescopes with a resolution the size of a continent, the Very Long Baseline Array (VLBA) employs observatories across the continental United States, Saint Croix in the U.S. Virgin Islands, and Mauna Kea, Hawaii. This is effectively the longest radio interferometer in the world with a baseline of over 8,600 kilometres and a resolution of under one milliarcseconds at 4 to 0.7 centimetre wavelengths. The VLBA has been used to study H2O megamasers in Active Galactic Nuclei and measure ultra-precise positions and proper motions of stars and galaxies.
LOFAR: Located just north of the town of Exloo in the Netherlands, The LOw Frequency Radio Array is a phased array 25,000 antennas with an effective collection area of 300,000 square metres. This makes LOFAR one of the largest single connected radio telescopes in existence. LOFAR is also a proof on concept for its eventual successor, the Square Kilometre Array to be built jointly in South Africa, Australia & New Zealand. Key projects involving LOFAR include extragalactic surveys, research into the nature of cosmic rays and studies of space weather.
The Pierre Auger Observatory: A cosmic ray observatory located in Malargüe, Argentina, the Pierre Auger Observatory was completed in 2008. This unique instrument consists of 1600 water tank Cherenkov radiation detectors spaced out over 3,000 square kilometres along with four complimenting fluorescence detectors. Results from Pierre Auger have thus far included discovery of a possible link between some of the highest energy events observed and active galactic nuclei.
GONG: Keeping an eye on the Sun is the goal of the Global Oscillation Network Group, a worldwide network of six solar telescopes. Established from an initial survey of 15 sites in 1991, GONG provides real-time data that compliments space-based efforts to monitor the Sun by the SDO, SHO, and STEREO A & B spacecraft. GONG scientists can even monitor the solar farside by use of helioseismology!
The Allen Telescope Array: Located at Hat Creek 470 kilometres northeast of San Francisco, this array will eventually consist of 350 Gregorian focus radio antennas that will support SETI’s search for extraterrestrial intelligence. 42 antennas were made operational in 2007, and a 2011 budget shortfall put the status of the array in limbo until a preliminary financing goal of $200,000 was met in August 2011.
The YBJ Cosmic Ray Observatory: Located high on the Tibetan plateau, Yangbajing International Cosmic Ray Observatory is a joint Japanese-Chinese effort. Much like Pierre-Auger, the YBJ Cosmic Ray Observatory employs scintillators spread out along with high speed cameras to watch for cosmic ray interactions. YBJ observes the sky in cosmic rays continuously and has captured sources from the Crab nebula pulsar and found a correlation between solar & interplanetary magnetic fields and the Sun’s own “cosmic ray shadow”. The KOSMA 3-metre radio telescope is also being moved from Switzerland to the YBJ observatory in Tibet.
Pulsar Jackpot Scours Old Data for New Discoveries
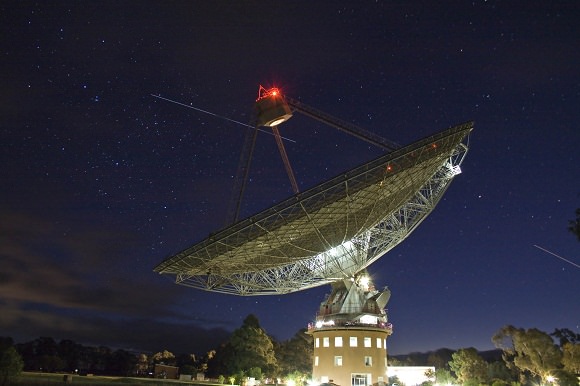
Chalk another one up for Citizen Science. Earlier this month, researchers announced the discovery of 24 new pulsars. To date, thousands of pulsars have been discovered, but what’s truly fascinating about this month’s discovery is that came from culling through old data using a new method.
A pulsar is a dense, highly magnetized, swiftly rotating remnant of a supernova explosion. Pulsars where first discovered by Jocelyn Bell Burnell and Antony Hewish in 1967. The discovery of a precisely timed radio beacon initially suggested to some that they were the product of an artificial intelligence. In fact, for a very brief time, pulsars were known as LGM’s, for “Little Green Men.” Today, we know that pulsars are the product of the natural death of massive stars.
The data set used for the discovery comes from the Parkes 64-metre radio observatory based out of New South Wales, Australia. The installation was the first to receive telemetry from the Apollo 11 astronauts on the Moon and was made famous in the movie The Dish. The Parkes Multi-Beam Pulsar Survey (PMPS) was conducted in the late 1990’s, making thousands of 35-minute recordings across the plane of the Milky Way galaxy. This survey turned up over 800 pulsars and generated 4 terabytes of data. (Just think of how large 4 terabytes was in the 90’s!)
The nature of these discoveries presented theoretical astrophysicists with a dilemma. Namely, the number of short period and binary pulsars was lower than expected. Clearly, there were more pulsars in the data waiting to be found.
Enter Citizen Science. Using a program known as Einstein@Home, researchers were able to sift though the recordings using innovative modeling techniques to tease out 24 new pulsars from the data.
“The method… is only possible with the computing resources provided by Einstein@Home” Benjamin Knispel of the Max Planck Institute for Gravitational Physics told the MIT Technology Review in a recent interview. The study utilized over 17,000 CPU core years to complete.
Einstein@Home is a program uniquely adapted to accomplish this feat. Begun in 2005, Einstein@Home is a distributed computing project which utilizes computing power while machines are idling to search through downloaded data packets. Similar to the original distributed computing program SETI@Home which searches for extraterrestrial signals, Einstein@Home culls through data from the LIGO (Laser Interferometer Gravitational Wave Observatory) looking for gravity waves. In 2009, the Einstein@Home survey was expanded to include radio astronomy data from the Arecibo radio telescope and later the Parkes observatory.
Among the discoveries were some rare finds. For example, PSR J1748-3009 Has the highest known dispersion measure of any millisecond pulsar (The dispersion measure is the density of free electrons observed moving towards the viewer). Another find, J1750-2531 is thought to belong to a class of intermediate-mass binary pulsars. 6 of the 24 pulsars discovered were part of binary systems.
These discoveries also have implications for the ongoing hunt for gravity waves by such projects as LIGO. Specifically, a through census of binary pulsars in the galaxy will give scientists a model for the predicted rate of binary pulsar mergers. Unlike radio surveys, LIGO seeks to detect these events via the copious amount of gravity waves such mergers should generate. Begun in 2002, LIGO consists of two gravity wave observatories, one in Hanford Washington and one in Livingston Louisiana just outside of Baton Rouge. Each LIGO detector consists of two 2 kilometre Fabry-Pérot arms in an “L” configuration which allow for ultra-precise measurements of a 200 watt laser beam shot through them. Two detectors are required to pin-point the direction of an incoming gravity wave on the celestial sphere. You can see the orientation of the “L’s” on the display on the Einstein@Home screensaver. Two geographically separate detectors are also required to rule out local interference. A gravity wave from a galactic source would ripple straight through the Earth.
Such a movement would be tiny, on the order of 1/1,000th the diameter of a proton, unnoticed by all except the LIGO detectors. To date, LIGO has yet to detect gravity waves, although there have been some false alarms. Scientists regularly interject test signals into the data to see if system catches them. The lack of detection of gravity waves by LIGO has put some constraints on certain events. For example, LIGO reported a non-detection of gravity waves during the February 2007 short gamma-ray burst event GRB 070201. The event arrived from the direction of the Andromeda Galaxy, and thus was thought to have been relatively nearby in the universe. Such bursts are thought to be caused by neutron star and/or black holes mergers. The lack of detection by LIGO suggests a more distant event. LIGO should be able to detect a gravitational wave event out to 70 million light years, and Advanced LIGO (AdLIGO) is set to go online in 2014 and will increase its sensitivity tenfold.
Knowledge of where these potential pulsar mergers are by such discoveries as the Parkes radio survey will also give LIGO researchers clues of targets to focus on. “The search for pulsars isn’t easy, especially for these “quiet” ones that aren’t doing the equivalent of “screaming” for our attention,” Says LIGO Livingston Data Analysis and EPO Scientist Amber Stuver. The LIGO consortium developed the data analysis technique used by Einstein@Home. The direct detection of gravitational waves by LIGO or AdLIGO would be an announcement perhaps on par with CERN’s discovery of the Higgs Boson last year. This would also open up a whole new field of gravitational wave astronomy and perhaps give new stimulus to the European Space Agencies’ proposed Laser Interferometer Space Antenna (LISA) space-based gravity wave detector. Congrats to the team at Parkes on their discovery… perhaps we’ll have the first gravity wave detection announcement out of LIGO as well in years to come!
-Read the original paper on the discovery of 24 new pulsars here.
-Amber Stuver blogs about Einstein@Home & the spin-off applications of gravity wave technology at Living LIGO.
-Parkes radio telescope image is copyrighted and used with the permission of CSIRO Operations Scientist John Sarkissian.
-For a fascinating read on the hunt for gravity waves, check out Gravity’s Ghost.