In 2013, the European Space Agency deployed the long-awaited Gaia space observatory. As one of a handful of next-generation space observatories that will be going up before the end of the decade, this mission has spent the past few years cataloging over a billion astronomical objects. Using this data, astronomers and astrophysicists hope to create the largest and most precise 3D map of the Milky Way to date.
Though it is almost to the end of its mission, much of its earliest information is still bearing fruit. For example, using the mission’s initial data release, a team of astrophysicists from the University of Toronto managed to calculate the speed at which the Sun orbits the Milky Way. From this, they were able to obtain a precise distance estimate between our Sun and the center of the galaxy for the first time.
For some time, astronomers have been unsure as to exactly how far our Solar System is from the center of our galaxy. Much of this has to do with the fact that it is impossible to view it directly, due to a combination of factors (i.e. perspective, the size of our galaxy, and visibility barriers). As a result, since the year 2000, official estimates have varied between 7.2 and 8.8 kiloparsecs (~23,483 to 28,700 light years).
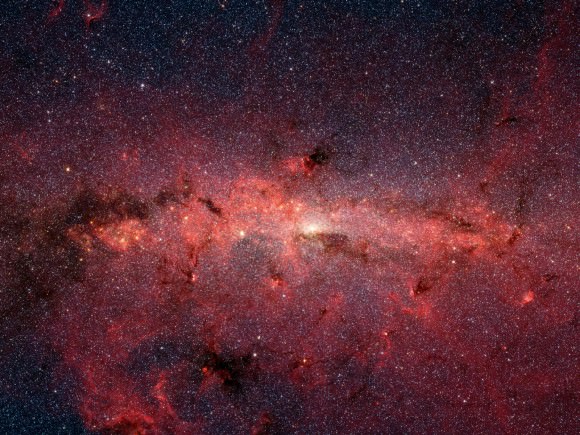
For the sake of their study, the team – which was led by Jason Hunt, a Dunlap Fellow at the Dunlap Institute for Astronomy & Astrophysics at the University of Toronto – combined Gaia’s initial release with data from the RAdial Velocity Experiment (RAVE). This survey, which was conducted between 2003 and 2013 by the Australian Astronomical Observatory (AAO), measured the positions, distances, radial velocities and spectra of 500,000 stars.
Over 200,000 of these stars were also observed by Gaia and information on them was included in its initial data release. As they explain in their study, which was published in the Journal of Astrophysical Letters in November 2016, they used this to examined the speeds at which these stars orbit the center of the galaxy (relative to the Sun), and in the process discovered that there was an apparent distribution in their relative velocities.
In short, our Sun moves around the center of the Milky Way at a speed of 240 km/s (149 mi/s), or 864,000 km/h (536,865 mph). Naturally, some of the more than 200,000 candidates were moving faster or slower. But for some, there was no apparent angular momentum, which they attributed to these stars being scattering onto “chaotic, halo-type orbits when they pass through the Galactic nucleus”.
As Hunt explained in Dunlap Institute press release:
“Stars with very close to zero angular momentum would have plunged towards the Galactic center where they would be strongly affected by the extreme gravitational forces present there. This would scatter them into chaotic orbits taking them far above the Galactic plane and away from the Solar neighbourhood… By measuring the velocity with which nearby stars rotate around our Galaxy with respect to the Sun, we can observe a lack of stars with a specific negative relative velocity. And because we know this dip corresponds to 0 km/sec, it tells us, in turn, how fast we are moving.”
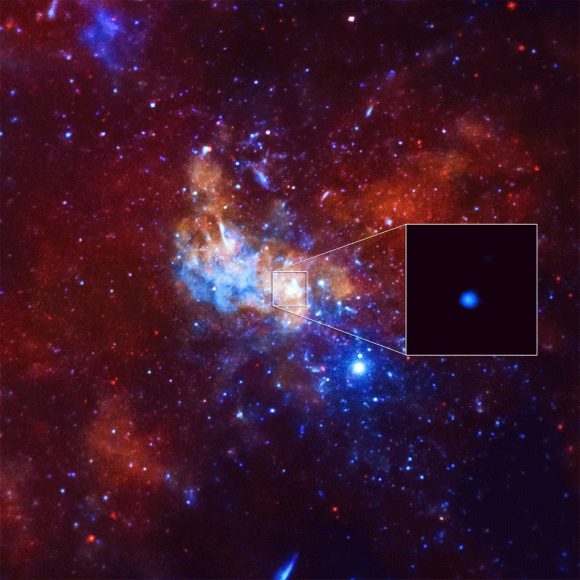
The next step was to combine this information with proper motion calculations of Sagittarius A* – the supermassive black hole believed to be at the center of our galaxy. After correcting for its motion relative to background objects, they were able to effectively triangulate the Earth’s distance from the center of the galaxy. From this, they derived a refined distance of estimate of 7.6 to 8.2 kpc – which works out to about 24,788 to 26,745 light years.
This study builds upon previous work conducted by the study’s co-authors – Prof. Ray Calberg, the current chair of the Department of Astronomy & Astrophysics at the University of Toronto. Years ago, he and Prof. Kimmo Innanen of the Department of Physics and Astronomy at York University conducted a similar study using radial velocity measurement from 400 of the Milky Way’s stars.
But by incorporating data from the Gaia observatory, the UofT team was able to obtain a much more comprehensive data set and narrow the distance to galactic center by a significant amount. And this was based on only the initial data released by the Gaia mission. Looking ahead, Hunt anticipates that further data releases will allow his team and other astronomers to refine their calculations even more.
“Gaia’s final release in late 2017 should enable us to increase the precision of our measurement of the Sun’s velocity to within approximately one km/sec,” he said, “which in turn will significantly increase the accuracy of our measurement of our distance from the Galactic center.”
As more next-generation space telescopes and observatories are deployed, we can expect them to provide us with a wealth of new information about our Universe. And from this, we can expect that astronomers and astrophysicists will begin to shine the light on a number of unresolved cosmological questions.
Further Reading: University of Toronto, The Astrophysical Journal Letters