When it comes to the many mysteries of the Universe, a special category is reserved for black holes. Since they are invisible to the naked eye, they remain visibly undetected, and scientists are forced to rely on “seeing” the effects their intense gravity has on nearby stars and gas clouds in order to study them.
That may be about to change, thanks to a team from Cardiff University. Here, researchers have achieved a breakthrough that could help scientists discover hundreds of black holes throughout the Universe.
Led by Dr. Mark Hannam from the School of Physics and Astronomy, the researchers have built a theoretical model which aims to predict all potential gravitational-wave signals that might be found by scientists working with the Laser Interferometer Gravitational-Wave Observatory (LIGO) detectors.
These detectors, which act like microphones, are designed to search out remnants of black hole collisions. When they are switched on, the Cardiff team hope their research will act as a sort of “spotters guide” and help scientists pick up the faint ripples of collisions – known as gravitational waves – that took place millions of years ago.
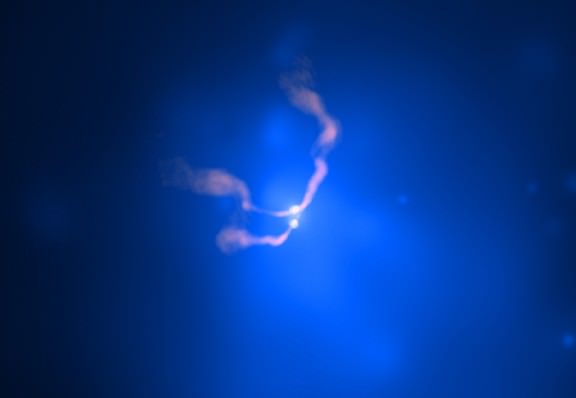
Made up of postdoctoral researchers, PhD students, and collaborators from universities in Europe and the United States, the Cardiff team will work with scientists across the world as they attempt to unravel the origins of the Universe.
“The rapid spinning of black holes will cause the orbits to wobble, just like the last wobbles of a spinning top before it falls over,” Hannam said. “These wobbles can make the black holes trace out wild paths around each other, leading to extremely complicated gravitational-wave signals. Our model aims to predict this behavior and help scientists find the signals in the detector data.”
Already, the new model has been programmed into the computer codes that LIGO scientists all over the world are preparing to use to search for black-hole mergers when the detectors switch on.
Dr Hannam added: “Sometimes the orbits of these spinning black holes look completely tangled up, like a ball of string. But if you imagine whirling around with the black holes, then it all looks much clearer, and we can write down equations to describe what is happening. It’s like watching a kid on a high-speed spinning amusement park ride, apparently waving their hands around. From the side lines, it’s impossible to tell what they’re doing. But if you sit next to them, they might be sitting perfectly still, just giving you the thumbs up.”
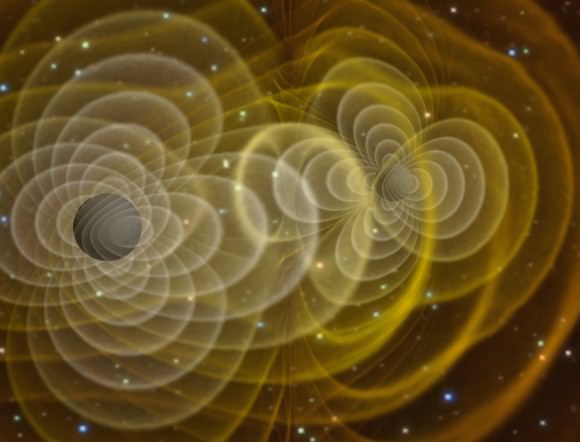
But of course, there’s still work to do: “So far we’ve only included these precession effects while the black holes spiral towards each other,” said Dr. Hannam. “We still need to work our exactly what the spins do when the black holes collide.”
For that they need to perform large computer simulations to solve Einstein’s equations for the moments before and after the collision. They’ll also need to produce many simulations to capture enough combinations of black-hole masses and spin directions to understand the overall behavior of these complicated systems.
In addition, time is somewhat limited for the Cardiff team. Once the detectors are switched on, it will only be a matter of time before the first gravitational wave-detections are made. The calculations that Dr. Hannam and his colleagues are producing will have to ready in time if they hope to make the most of them.
But Dr. Hannam is optimistic. “For years we were stumped on how to untangle the black-hole motion,” he said. “Now that we’ve solved that, we know what to do next.”
Further Reading: News Center – Cardiff U