For decades, we have seen Mars as a desolate landscape devoid of any signs of life. Attempt to identify ways of growing plants and food on the red planet have focussed on greenhouse like structures to enable plants to survive, that is, until now! A desert moss called ‘Syntrichia caninervis’ has been identified and it can grown in extreme environments like Antarctica and the Mojave Desert. A new study revealed the moss can survive Mars-like environments too including low temperatures, high levels of radiation and drought.
Continue reading “The Rugged Desert Moss Best Equipped to Survive on Mars”Want to Start a Farm on Mars? This Rover Will Find Out if it’s Possible
Travelling to Mars has its own challenges. The distance alone makes the journey something of a mission in itself. Arrive though, and the handwork has only just begun. Living and surviving on Mars will be perhaps humans biggest challenge yet. It would be impossible to take everything along with you to survive so instead, it would be imperative to ‘live off the land’ and produce as much locally as possible. A new rover called AgroMars will be equipped with a number of agriculture related experiments to study the make up of the soil to assess its suitability for growing food.
Continue reading “Want to Start a Farm on Mars? This Rover Will Find Out if it’s Possible”Should We Send Humans to Mars?
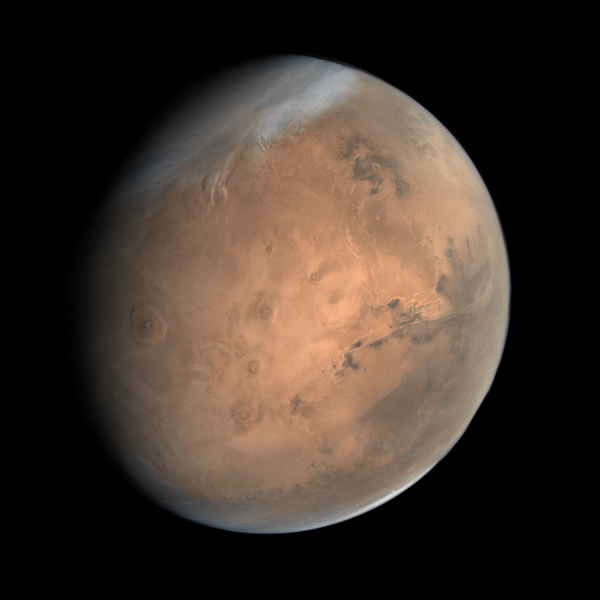
Universe Today has explored the potential for sending humans to Europa, Venus, Titan, and Pluto, all of which possess environmental conditions that are far too harsh for humans to survive. The insight gained from planetary scientists resulted in some informative discussions, and traveling to some of these far-off worlds might be possible, someday. In the final installment of this series, we will explore the potential for sending humans to a destination that has been the focus of scientific exploration and science folklore for more than 100 years: Mars aka the Red Planet.
Continue reading “Should We Send Humans to Mars?”NASA is Pushing Back its Moon Landings to 2026
I wasn’t around for the Apollo program that took human beings to the Moon. I would have love to have seen it all unfold though. With NASAs Artemis program the opportunity will soon be with us again to watch humans set foot on another world, just not for the first time. Alas NASA announced on Tuesday that the Moon landings which form part of Artemis 3, have been pushed back one year to 2026.
Continue reading “NASA is Pushing Back its Moon Landings to 2026”Space and Sustainability: How the Lessons of Biosphere 2 Inspired SAM²
A lot has been said, penned, and documented about the famous experiment known as “Biosphere 2” (B2). For anyone whose formative years coincided with the early 90s, this name probably sounds familiar. Since the project launched in 1991, it has been heavily publicized, criticized, and was even the subject of a documentary – titled “Spaceship Earth” – that premiered in May of 2020.
To listen to some of what’s been said about B2 (even after 30 years), one might get the impression that it was a failure that proved human beings cannot live together in a sealed environment for extended periods of time. But in truth, it was a tremendous learning experience, the results of which continue to inform human spaceflight and ecosystem research today. In an era of renewed interplanetary exploration, those lessons are more vital than ever.
This is the purpose behind the Space Analog for the Moon and Mars (SAM²), a new analog experiment led by Kai Staats and John Adams. Along with an international team of specialists, experts from the University of Arizona, and support provided by NASA, the National Geographic Society, and commercial partners, SAM² will validate the systems and technology that will one-day allow for colonies on the Moon, Mars, and beyond.
Continue reading “Space and Sustainability: How the Lessons of Biosphere 2 Inspired SAM²”Chitin Could be the Perfect Building Material on Mars
It’s hard to deny that we’re heading for a future with a human presence on Mars. But to develop sustained presence, there are an enormous number of technical problems to be worked out. One of those problems concerns manufacturing and building.
We can’t send everything people will need to Mars. We’ll need some way to build structures, and tools and other things.
Continue reading “Chitin Could be the Perfect Building Material on Mars”Learning to Live Sustainably on the Red Planet: Habitat Mars
There’s quite a bit of buzz these days about how humanity could become a “multiplanetary” species. This is understandable considering that space agencies and aerospace companies from around the world are planning on conducting missions to Low Earth Orbit (LEO), the Moon, and Mars in the coming years, not to mention establishing a permanent human presence there and beyond.
To do this, humanity needs to develop the necessary strategies for sustainable living in hostile environments and enclosed spaces. To prepare humans for this kind of experience, groups like Habitat Marte (Mars Habitat) and others are dedicated to conducting simulated missions in analog environments. The lessons learned will not only prepare people to live and work in space but foster ideas for sustainable living here on Earth.
Continue reading “Learning to Live Sustainably on the Red Planet: Habitat Mars”Ideas for Sustainable Cities and Urban Farming… on Mars?
“The core essence of Mars City Design is —not to repeat the same mistakes that we did to our planet. The hope to start a new design for living on Mars, every single thing needs to have a sustainable answer within the big picture, of the regenerative circle of life and of the product itself. All things need an exit plan that allows them to be reusable or repurposed. That can hopefully inspire change on Earth.”
-Vera Mulyani (Vera Mars), Founder/CEO Mars City Design
Once the stuff of science fiction, the possibility that humans could establish a permanent settlement on Mars now appears to be a genuine possibility. While doing so represents a major challenge and there are many hurdles that still need to be overcome, the challenge itself is inspiring some truly creative solutions. But what is especially interesting is how these same solutions can also address problems here on Earth.
This is especially clear where the Mars City Design Challenges are concerned. This annual competition was founded with the purpose of inspiring innovative ideas that could lead to sustainable living on Mars. For this year’s challenge, “Urban Farming for Extreme Environment,” Mars City Design and its founder (Vera Mulyani) are looking for designs that incorporate urban farming to support a colony of 100 people.
Continue reading “Ideas for Sustainable Cities and Urban Farming… on Mars?”Want To Explore Mars? Send Humans To The Moons Of Mars First: Phobos And Deimos
Humans to Mars. That’s the plan right? The problem is that sending humans down to the surface of Mars is one of the most complicated and ambitious goals that we can attempt. It’s a huge step to go from low Earth orbit, then lunar landings, and then all the way to Mars, a journey of hundreds of millions of kilometers and 2 years at the least.
Continue reading “Want To Explore Mars? Send Humans To The Moons Of Mars First: Phobos And Deimos”Five Teams Compete to Design a 3D Printed Mars Habitat for NASA

If and when we decide to go to Mars (and stay there), the Martian settlers will face some serious challenges. For one, the planet is extremely cold compared to Earth, averaging at about -63 °C (-82°F), which is comparable to cold night in Antarctica. On top of that, there’s the incredibly thin atmosphere that is unbreathable to humans and terrestrial creatures. Add to that the radiation, and you begin to see why settling Mars will be difficult.
But as the saying goes, necessity is the mother of invention. And to stimulate the invention process, NASA has partnered with Bradley University of Peoria to launch the 3D-Printed Habitat Centennial Challenge competition. As part of NASA’s Centennial Challenges, which are sponsored by the Space Technology Mission Directorate, this competition recently awarded $100,000 in prize money to five teams for their design concepts.
The NASA Centennial Challenges were initiated in 2005 to directly engage the public, and produce revolutionary applications for space exploration challenges. The program offers incentive prizes to stimulate innovation in basic and applied research, technology development, and prototype demonstration. To administer the competition, Bradley University also partnered with sponsors Caterpillar, Bechtel and Brick & Mortar Ventures.
For the competition, participants were tasked with creating digital representations of the physical and functional characteristics of a Martian habitat using specialized software tools. A panel of NASA, academic and industry experts awarded the team points based on various criteria, which determined how much prize money each winning team got. Out of 18 submissions from all over the world, 5 teams were selected.
In order of how much prize money they were awarded, the winning teams were:
- Team Zopherus of Rogers, Arkansas – $20,957.95
- AI. SpaceFactory of New York – $20,957.24
- Kahn-Yates of Jackson, Mississippi – $20,622.74
- SEArch+/Apis Cor of New York – $19,580.97
- Northwestern University of Evanston, Illinois – $17,881.10
The design competition emphasizes all the challenges that building a life-supporting habitat on Mars would entail, which includes the sheer distances involved and the differences in atmosphere and landscapes. In short, the teams needed to create habitats that would be insulated and air-tight and could also be built using local materials (aka. in-situ resource utilization).
The competition began in 2014 and has been structured in three phases. For Phase 1, the Design Competition (which was completed in 2015 with $50,000 prize purse), the teams were required to submit a rendering of their proposed habitat. Phase 2, the Structural Member Competition, focused on material technologies and required teams to create structural components. This phase was completed in 2017 with a $1.1 million prize purse.
For Phase 3, the On-Site Habitat Competition – which is the current phase of the competition – competitors were tasked with fabricated sub-scale versions of their habitats. This phase has five levels of competition, which consist of two virtual levels and three construction levels. For the former, the teams were tasked with using Building Information Modeling (BIM) software to design a habitat that combines all the structural requirements and systems it must contain.
For the construction levels, the teams will be required to autonomously fabricate 3D-printed elements of the habitat, culminating with a one-third-scale printed habitat for the final level. By the end of this phase, teams will be awarded prize money from a $2 million purse. As Monsi Roman, the program manager for NASA’s Centennial Challenges, said in a recent NASA press statement:
“We are thrilled to see the success of this diverse group of teams that have approached this competition in their own unique styles. They are not just designing structures, they are designing habitats that will allow our space explorers to live and work on other planets. We are excited to see their designs come to life as the competition moves forward.”
The winning entries included team Zorphues’ concept for a modular habitat that was inspired by biological structures here on Earth. The building-process begins with a lander (which is also a mobile print factory) reaching the surface and scanning the environment to find a good “print area”. It then walks over this area and deploys rovers to gather materials, then seals to the ground to provide a pressurized print environment.
The main module is then assembled using pre-fabricated components (like airlocks, windows, atmospheric control, toilets, sinks, etc), and the structure is printed around it. The printer then walks itself to an adjacent location, and prints another module using the same method. In time, a number of habitats are connected to the main module that provide spaces for living, recreation, food production, scientific studies, and other activities.
For their concept, the second place team (Team AI. SpaceFactory) selected a vertically-oriented cylinder as the most efficient shape for their Marsha habitat. According to the team, this design is not only the ideal pressure environment, but also maximizes the amount of usable space, allows for the structure to be vertically-divided based on activities, is well-suited to 3-D printing and takes up less surface space.
The team’s also designed their habitat to deal with temperature changes on Mars, which are significant. Their solution was to design the entire structure as a flanged shell that moves on sliding bearings at its foundation in response to temperature changes. The structure is also a double shell, with the outer (pressure) shell separate from the inner habitat entirely. This optimizes air flow and allows for light to filters in to the entire habitat.
Next up is the Khan-Yates habitat, which the team designed to be specifically-suited to withstand dust storms and harsh climates on the Red Planet. This coral-like dome consists of a lander that would set down in the equatorial region, then print a foundation and footing layer using local materials. The print arm would then transition vertically to begin printing the shell and the floors.
The outer shell is studded with windows that allow for a well-lit environment, the outer shell is separate from the core, and the shape of the structure is designed to ensure that dust storms flow around the structure. In fourth place was SEArch+/Apis Cor’s Mars X house, a habitat designed to provide maximum radiation protection while also ensuring natural light and connections to the Martian landscape.
The habitat is constructed by mobile robotic printers, which are deployed from a Hercules Single-Stage Reusable Lander. The design is inspired by Nordic architecture, and uses “light scoops” and floor-level viewing apertures to ensure that sunlight in the northern latitudes makes it into the interior. The two outer (and overlapping) shells house the living areas, which consist of two inflatable spaces with transparent CO2 inflated window pockets.
Fifth place went to the team from Northwestern University for their Martian 3Design habitat, which consists of an inner sphere closed-shell and an outer parabolic dome. According to the team, this habitat provides protection from the Martian elements through three design features. The first is the internal shape of the structure, which consists of a circular foundation, an inflatable pressure vessel that serves as the main living area, and the outer shell.
The second feature is the entryway system, which extend from opposite ends of the structure and serves as entrances and exits and could provide junctions with future pods. The third feature is the cross-beams that are the structural backbone of the dome and are optimized for pressure-loading under Martian gravity and atmospheric conditions, and provide continuous protection from radiation and the elements.
The interior layout is based on the NASA Hawai’i Space Exploration Analog and Simulation (HI-SEAS) habitat, and is divided between “wet areas” and “dry areas”. These areas are placed on opposite sides of the habitat to optimize the use of resources by concentrated in them on one side (rather than have them running throughout that habitat), and space is also divided by a central, retractable wall that separates the interior into public and private areas.
Together, these concepts embody the aims of the 3D-Printed Habitat Centennial Challenge, which is to harness the talents of citizen inventors to develop the technologies necessary to build sustainable shelters that will one-day allow humans to live on the Moon, Mars and beyond. As Lex Akers, dean of the Caterpillar College of Engineering and Technology at Bradley University, said of the competition:
“We are encouraging a wide range of people to come up with innovative designs for how they envision a habitat on Mars. The virtual levels allow teams from high schools, universities and businesses that might not have access to large 3D printers to still be a part of the competition because they can team up with those who do have access to such machinery for the final level of the competition.”
Carrying on in the tradition of the Centennial Prizes, NASA is seeking public engagement with this competition to promote interest in space exploration and address future challenges. It also seeks to leverage new technologies in order to solve the many engineering, technical and logistical problems presented by space travel. Someday, if and when human beings are living on the Moon, Mars, and other locations in the Solar System, the habitats they call home could very well be the work of students, citizen inventors and space enthusiasts.
For more information on the 3-D Pinrted Habitat Challenge, check out the competition web page.
Further Reading: NASA