The early 20th century was a very auspicious time for the sciences. In addition to Ernest Rutherford and Niels Bohr giving birth to the Standard Model of particle physics, it was also a period of breakthroughs in the field of quantum mechanics. Thanks to ongoing studies on the behavior of electrons, scientists began to propose theories whereby these elementary particles behaved in ways that defied classical, Newtonian physics.
One such example is the Electron Cloud Model proposed by Erwin Schrodinger. Thanks to this model, electrons were no longer depicted as particles moving around a central nucleus in a fixed orbit. Instead, Schrodinger proposed a model whereby scientists could only make educated guesses as to the positions of electrons. Hence, their locations could only be described as being part of a ‘cloud’ around the nucleus where the electrons are likely to be found.
Atomic Physics To The 20th Century:
The earliest known examples of atomic theory come from ancient Greece and India, where philosophers such as Democritus postulated that all matter was composed of tiny, indivisible and indestructible units. The term “atom” was coined in ancient Greece and gave rise to the school of thought known as “atomism”. However, this theory was more of a philosophical concept than a scientific one.

It was not until the 19th century that the theory of atoms became articulated as a scientific matter, with the first evidence-based experiments being conducted. For example, in the early 1800’s, English scientist John Dalton used the concept of the atom to explain why chemical elements reacted in certain observable and predictable ways. Through a series of experiments involving gases, Dalton went on to develop what is known as Dalton’s Atomic Theory.
This theory expanded on the laws of conversation of mass and definite proportions and came down to five premises: elements, in their purest state, consist of particles called atoms; atoms of a specific element are all the same, down to the very last atom; atoms of different elements can be told apart by their atomic weights; atoms of elements unite to form chemical compounds; atoms can neither be created or destroyed in chemical reaction, only the grouping ever changes.
Discovery Of The Electron:
By the late 19th century, scientists also began to theorize that the atom was made up of more than one fundamental unit. However, most scientists ventured that this unit would be the size of the smallest known atom – hydrogen. By the end of the 19th century, his would change drastically, thanks to research conducted by scientists like Sir Joseph John Thomson.
Through a series of experiments using cathode ray tubes (known as the Crookes’ Tube), Thomson observed that cathode rays could be deflected by electric and magnetic fields. He concluded that rather than being composed of light, they were made up of negatively charged particles that were 1ooo times smaller and 1800 times lighter than hydrogen.
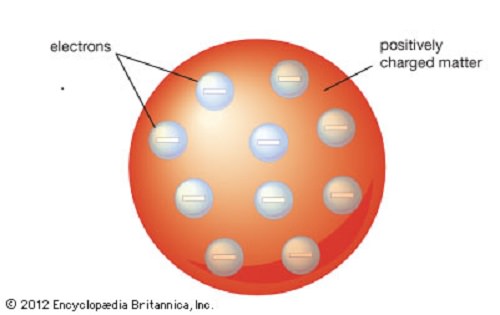
This effectively disproved the notion that the hydrogen atom was the smallest unit of matter, and Thompson went further to suggest that atoms were divisible. To explain the overall charge of the atom, which consisted of both positive and negative charges, Thompson proposed a model whereby the negatively charged “corpuscles” were distributed in a uniform sea of positive charge – known as the Plum Pudding Model.
These corpuscles would later be named “electrons”, based on the theoretical particle predicted by Anglo-Irish physicist George Johnstone Stoney in 1874. And from this, the Plum Pudding Model was born, so named because it closely resembled the English desert that consists of plum cake and raisins. The concept was introduced to the world in the March 1904 edition of the UK’s Philosophical Magazine, to wide acclaim.
Development Of The Standard Model:
Subsequent experiments revealed a number of scientific problems with the Plum Pudding model. For starters, there was the problem of demonstrating that the atom possessed a uniform positive background charge, which came to be known as the “Thomson Problem”. Five years later, the model would be disproved by Hans Geiger and Ernest Marsden, who conducted a series of experiments using alpha particles and gold foil – aka. the “gold foil experiment.”
In this experiment, Geiger and Marsden measured the scattering pattern of the alpha particles with a fluorescent screen. If Thomson’s model were correct, the alpha particles would pass through the atomic structure of the foil unimpeded. However, they noted instead that while most shot straight through, some of them were scattered in various directions, with some going back in the direction of the source.

Geiger and Marsden concluded that the particles had encountered an electrostatic force far greater than that allowed for by Thomson’s model. Since alpha particles are just helium nuclei (which are positively charged) this implied that the positive charge in the atom was not widely dispersed, but concentrated in a tiny volume. In addition, the fact that those particles that were not deflected passed through unimpeded meant that these positive spaces were separated by vast gulfs of empty space.
By 1911, physicist Ernest Rutherford interpreted the Geiger-Marsden experiments and rejected Thomson’s model of the atom. Instead, he proposed a model where the atom consisted of mostly empty space, with all its positive charge concentrated in its center in a very tiny volume, that was surrounded by a cloud of electrons. This came to be known as the Rutherford Model of the atom.
Subsequent experiments by Antonius Van den Broek and Niels Bohr refined the model further. While Van den Broek suggested that the atomic number of an element is very similar to its nuclear charge, the latter proposed a Solar-System-like model of the atom, where a nucleus contains the atomic number of positive charge and is surrounded by an equal number of electrons in orbital shells (aka. the Bohr Model).
The Electron Cloud Model:
During the 1920s, Austrian physicist Erwin Schrodinger became fascinated by the theories Max Planck, Albert Einstein, Niels Bohr, Arnold Sommerfeld, and other physicists. During this time, he also became involved in the fields of atomic theory and spectra, researching at the University of Zurich and then the Friedrich Wilhelm University in Berlin (where he succeeded Planck in 1927).
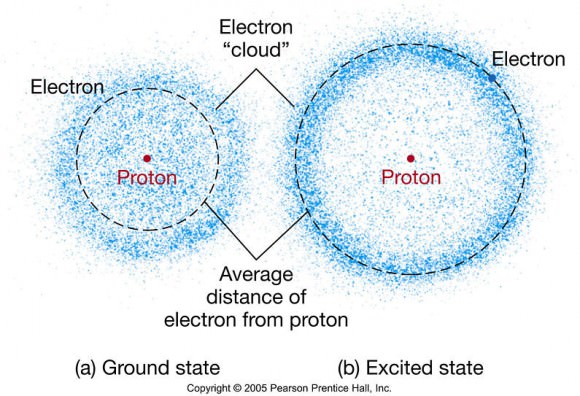
In 1926, Schrödinger tackled the issue of wave functions and electrons in a series of papers. In addition to describing what would come to be known as the Schrodinger equation – a partial differential equation that describes how the quantum state of a quantum system changes with time – he also used mathematical equations to describe the likelihood of finding an electron in a certain position.
This became the basis of what would come to be known as the Electron Cloud (or quantum mechanical) Model, as well as the Schrodinger equation. Based on quantum theory, which states that all matter has properties associated with a wave function, the Electron Cloud Model differs from the Bohr Model in that it does not define the exact path of an electron.
Instead, it predicts the likely position of the location of the electron based on a function of probabilities. The probability function basically describes a cloud-like region where the electron is likely to be found, hence the name. Where the cloud is most dense, the probability of finding the electron is greatest; and where the electron is less likely to be, the cloud is less dense.
These dense regions are known as “electron orbitals”, since they are the most likely location where an orbiting electron will be found. Extending this “cloud” model to a 3-dimensional space, we see a barbell or flower-shaped atom (as in image at the top). Here, the branching out regions are the ones where we are most likely to find the electrons.
Thanks to Schrodinger’s work, scientists began to understand that in the realm of quantum mechanics, it was impossible to know the exact position and momentum of an electron at the same time. Regardless of what the observer knows initially about a particle, they can only predict its succeeding location or momentum in terms of probabilities.
At no given time will they be able to ascertain either one. In fact, the more they know about the momentum of a particle, the less they will know about its location, and vice versa. This is what is known today as the “Uncertainty Principle”.
Note that the orbitals mentioned in the previous paragraph are formed by a hydrogen atom (i.e. with just one electron). When dealing with atoms that have more electrons, the electron orbital regions spread out evenly into a spherical fuzzy ball. This is where the term ‘electron cloud’ is most appropriate.
This contribution was universally recognized as being one of the cost important contributions of the 20th century, and one which triggered a revolution in the fields of physics, quantum mechanics and indeed all the sciences. Thenceforth, scientists were no longer working in a universe characterized by absolutes of time and space, but in quantum uncertainties and time-space relativity!
We have written many interesting articles about atoms and atomic models here at Universe Today. Here’s What Is John Dalton’s Atomic Model?, What Is The Plum Pudding Model?, What Is Bohr’s Atomic Model?, Who Was Democritus?, and What Are The Parts Of An Atom?
For more information, be sure to check What Is Quantum Mechanics? from Live Science.
Astronomy Cast also has episode on the topic, like Episode 130: Radio Astronomy, Episode 138: Quantum Mechanics, and Episode 252: Heisenberg Uncertainty Principle