How is the James Webb Space Telescope different than the Hubble Space Telescope? What will JWST be looking for when it begins operating? In this short video, NASA astrophysicist Dr. Amber Straughn answers questions, and offers facts and images to explain what the Webb Space Telescope will tell us about the cosmos.
JWST Sunscreen Offers SPF 1,000,000
The James Webb Space Telescope will have a sunshield that is about the size of a tennis court, and mission managers say it will offer the best “SPF” (Sun Protection Factor) in the Universe.
“Each of the five layers of the shield is less than half the thickness of a piece of paper,” said John Durning, Deputy Project Manager for JWST. “The five work together to create an effective SPF of 1,000,000.”
This sunshield protects the observatory from unwanted light, keeping it cool and allowing it to detect heat from faraway objects in the universe. So, how do you get something that large into orbit?
Continue reading “JWST Sunscreen Offers SPF 1,000,000”
Costs for James Webb Telescope Soar — Again
[/caption]
The price tag for NASA’s next big space telescope keeps rising and the launch date will likely be delayed as well. A new report from an independent panel on the James Webb Space Telescope reveals it will take about $6.5 billion to launch and run the telescope for its projected 10-year mission. The price had previously ballooned from $3.5 billion to $5 billion. Originally the telescope was slated to launch in 2007, but was pushed back to 2014. Now, the panel says, the earliest launch date would be in September 2015.
The panel, requested by Congress, said there appears to be no technical issues with the telescope, but budget and management problems are the reasons for the cost overruns and delays.
“There is no reason to question the technical integrity of the design or of the team’s ability to deliver a quality product to orbit,” said John Casani from the Jet Propulsion Lab, who chaired the panel. “The problems causing cost growth and schedule delays have been associated with budgeting and program management, not technical performance.”
The money to cover the overruns will require $250 million more in NASA’s FY 2011 and 2012 budget. But with the current state of affairs in the country and Congress, it is likely other programs will suffer or be cut in order to pay for JWST.
In a teleconference with reporters, NASA associate administrator Chris Scolese admitted that NASA officials did not do a very good job of keeping track of what was going on with the massive telescope project.
“We were missing a certain fraction of what was going on,” Scolese. “The fault lies with us.”
The panel concluded that the budget was not sufficient in the early days of the telescope’s development for everything to go as hoped.
“The budget was flawed, from a money standpoint it was just insufficient to carry out the work,” said John Klineberg, a member of the panel and a retired engineer. “The budget was skewed, and the reserves to complete the work were also wrong because they were predicated on a budget that was too low. Headquarters did not spot the errors, and they didn’t fully recognize the extent to which the budget was understating the needs of the project.”
“This is a large, complex project and to estimate something to a real degree of precision is hard,” Klineberg added.
The panel found no way for current costs to be reduced, but found ways to reduce the likelihood of cost-growth in the future.
In order for JWST to be built and launched, the panel said NASA should restructure the project organization at Goddard Spaceflight Center to improve the accounting of costs and reserves. The program will now report directly to the Administrator’s office. Richard Howard will be the new JWST program director, replacing Phil Sabelhaus.
“We have to focus on doing what is right to get the project back on track,” said Scolese, “but I want to emphasize that there are no technical problems with the telescope and we have to thank the team for doing a great technical job. The important thing we have to fix is the cost management at the project level and at the management level.”
In a statement, NASA Administrator Charlie Bolden said, “I am disappointed we have not maintained the level of cost control we strive to achieve, something the American taxpayer deserves in all of our projects….NASA is committed to finding a sustainable path forward for the program based on realistic cost and schedule assessments.”
The teleconference with journalists included a first – at least for this reporter: one caller berated NASA management and swore at Scolese, obviously frustrated by the lack of oversight by NASA on what is supposed to be a flagship mission for the space agency’s astronomy division.
The infrared telescope will have a 6.5 meter (22 ft.) mirror and a sunshade the size of a tennis court. JWST should be able to look back in time to find the first galaxies that formed in the early Universe, and to peer inside dust clouds where stars and planetary systems are forming.
Read the report (pdf): James Webb Space Telescope Independent Comprehensive Review Panel — Nov. 10, 2010
JWST Built with ‘Unobtainium’
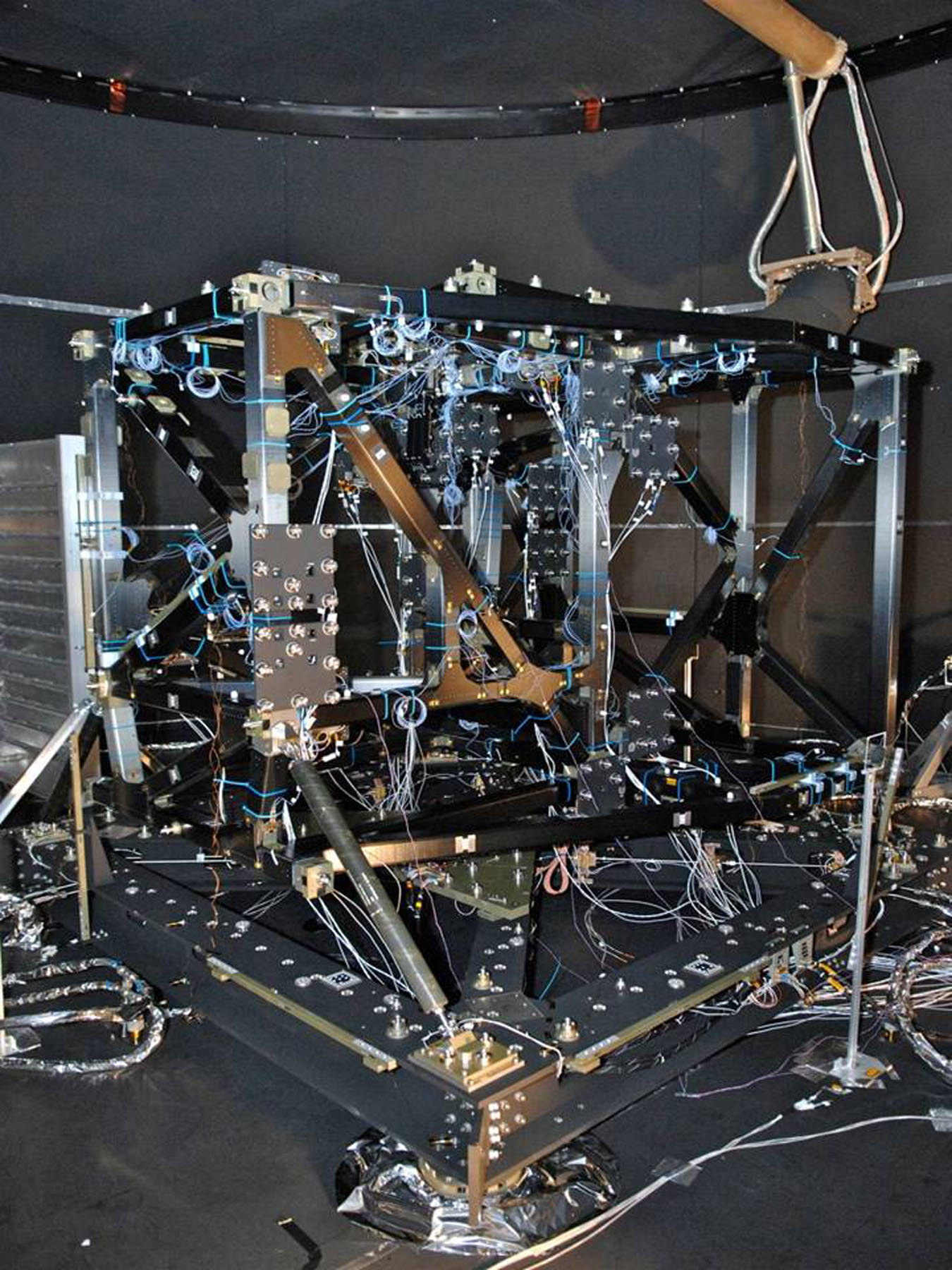
[/caption]
The James Webb Space Telescope (JWST) is the much anticipated, long awaited “next generation” telescope, which we hope will look further back in time, and deeper within dusty star forming regions, using longer wavelengths and more sensitivity than any previous space telescope. In order to take us to this next level, you’d kinda figure that new technologies would have to be developed in order for this ground-breaking, super-huge telescope to be built. You’d be right.
In fact, engineers had to use a little unobtainium to build the one-of-a-kind chassis, the backbone that will hold the spacecraft together.
Unobtainium isn’t just the name of the material mined in James Cameron’s movie “Avatar.” It is a word used in engineering — and sometimes fiction – to describe any extremely rare, costly, or physically impossible material or device needed to fulfill a given design for a given application.
The chassis for JWST – called the the Integrated Science Instrument Module ISIM – is made of a never-before-manufactured composite material which had to withstand the super-cold temperatures it will encounter when the observatory reaches its orbit 1.5-million kilometers (930,000 miles) from Earth.
The ISIM just passed an extremely important test, surviving temperatures that plunged as low as 27 Kelvin (-411 degrees Fahrenheit), colder than the surface of Pluto during a cycle of testing in Goddard’s Space Environment Simulator — a three-story thermal-vacuum chamber that simulates the temperature and vacuum conditions found in space.
The team at Goddard Space Flight Center who were charged with building the chassis needed a material that would assure the various instruments on JWST would maintain a precise cryogenic alignment and stability, yet survive the extreme gravitational forces experienced during launch.
The test was done to find out whether the car-sized structure contracted and distorted as predicted when it cooled from room temperature to the frigid — very important since the science instruments must maintain a specific location on the structure to receive light gathered by the telescope’s 6.5-meter (21.3-feet) primary mirror. If the structure shrunk or distorted in an unpredictable way due to the cold, the instruments no longer would be in position to gather data about everything from the first luminous glows following the Big Bang to the formation of star systems capable of supporting life.
When they first began, there was nothing out there that remotely fit the description of what was needed. So, that left one alternative: developing their own as-yet-to-be manufactured material, which team members jokingly referred to as “unobtainium.” Through mathematical modeling, the team discovered that by combining two composite materials, it could create a carbon fiber/cyanate-ester resin system that would be ideal for fabricating the structure’s square tubes that measure 75-mm (3-inch) in diameter.
During the recent 26-day test, and with repeated cycles of testing, the truss-like assembly designed by Goddard engineers did not crack. The structure shrunk as predicted by only 170 microns — the width of a needle —when it reached 27 Kelvin (-411 degrees Fahrenheit), far exceeding the design requirement of about 500 microns. “We certainly wouldn’t have been able to realign the instruments on orbit if the structure moved too much,” said ISIM Structure Project Manager Eric Johnson. “That’s why we needed to make sure we had designed the right structure.”
This type of structure could serve NASA in the future for the next-generation beyond JWST, and could also be a “spinoff” that manufacturers could find useful in designing structures that demand a high tolerance in conditions.
Source: NASA Goddard
Extrasolar Volcanoes May Soon be Detectable
[/caption]
We’ve all seen pictures of erupting terrestrial volcanoes from space, and even eruptions on Jupiter’s moon Io in the outer solar system, but would it be possible to detect an erupting volcano on an exoplanet? Astronomers say the answer is yes! (with a few caveats)
It’s going to be decades before telescopes will be able to resolve even the crudest surface features of rocky extrasolar planets, so don’t hold your breath for stunning photos of alien volcanoes outside our solar system. But astronomers have already been able to use spectroscopy to detect the composition of exoplanet atmospheres, and a group of theorists at the Harvard-Smithsonian Center for Astrophysics think a similar technique could detect the atmospheric signature of exo-eruptions.
By collecting spectra right before and right after the planet goes behind its star, astronomers can subtract out the star’s spectrum and isolate the signal from the planet’s atmosphere. Once this is done, they can look for evidence of molecules common in volcanic eruptions. Models suggest that sulfur dioxide is the best candidate for detection because volcanoes produce it in huge quantities and it lasts in a planet’s atmosphere for a long time.
Still, it won’t be easy.
“You would need something truly earthshaking, an eruption that dumped a lot of gases into the atmosphere,” said Smithsonian astronomer Lisa Kaltenegger. “Using the James Webb Space Telescope, we could spot an eruption 10 to 100 times the size of Pinatubo for the closest stars,” she added.

In 1991 Mount Pinatubo in the Philippines belched 17 million tons of sulfur dioxide into the stratosphere. Volcanic eruptions are ranked using the Volcanic Explosivity Index (VEI). Pinatubo ranked ‘colossal’ (VEI of 6) and the largest eruption in recorded history was the ‘super-colossal’ Tambora event in 1815. With a VEI of 7 it was about 10 times as large as Pinatubo. Even larger eruptions (more than 100 times larger than Pinatubo) on Earth are not unheard of: geologic evidence suggests that there have been 47 such eruptions in the past 36 million years, including the eruption of the Yellowstone caldera about 600,000 years ago.
The best candidates for detecting extrasolar volcanoes are super-earths orbiting nearby, dim stars, but the Kaltenegger and her colleagues found that volcanic gases on any earth-like planet up to 30 light years away might be detectable. Now they just have to wait until the James Webb Space Telescope is launched 2014 to test their prediction.
Sexy New James Webb Space Telescope “Trailer”
In anticipation of its launch in 2014, and perhaps in response to the Hubble3-D IMAX movie, the James Webb Space Telescope folks have put together this impressive preview of things to come. If you really want to pump it up, watch in in HD (mouse-over the little box on the bottom right that says 360.)
Continue reading “Sexy New James Webb Space Telescope “Trailer””
JWST Will Provide Capability to Search for Biomarkers on Earth-like Worlds
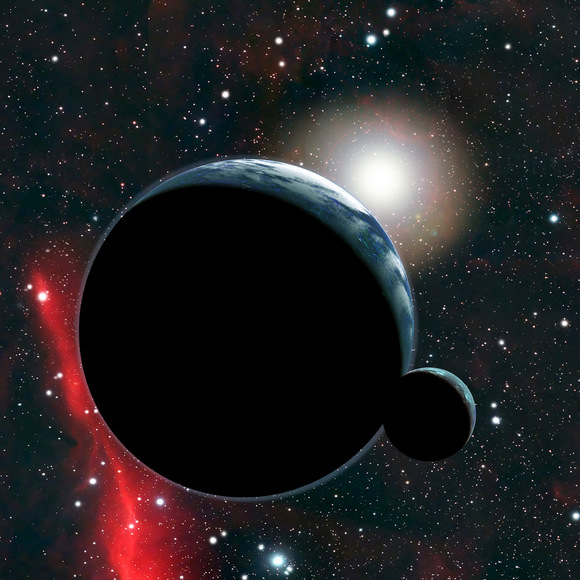
[/caption]
Does another Earth exist somewhere in our galaxy? With the recent lauch of the Kepler spacecraft, astronomers are getting closer and closer to finding an Earth-sized planet in an Earth-like orbit. But once that search succeeds, the next questions driving research will be: Is that planet habitable? Does it have an Earth-like atmosphere? Answering those questions will not be easy. But the telescope up for the task is the James Webb Space Telescope (JWST), set for a planned launch in 2013. Two researchers recently examined the ability of JWST to characterize the atmospheres of hypothetical Earth-like planets, and found this is the telescope that would be able to detect certain gases called biomarkers, such as ozone and methane, for close Earth-size worlds. (See our related article: Q&A with Dr. John Mather on JWST.)
Due to its large mirror and location at the L2 point in outer space, the James Webb Space Telescope will offer astronomers the first real possibility of finding the answers about the habitability of nearby Earth-like worlds, say Lisa Kaltenegger from the Harvard-Smithsonian Center for Astrophysics and Wesley Traub from the Jet Propulsion Laboratory. “We’ll have to be really lucky to decipher an Earth-like planet’s atmosphere during a transit event so that we can tell it is Earth-like,” said Kaltenegger. “We will need to add up many transits to do so – hundreds of them, even for stars as close as 20 light-years away.”
“Even though it’s hard, it will be an incredibly exciting endeavor to characterize a distant planet’s atmosphere,” she added.
In a transit event, a distant, extrasolar planet crosses in front of its star as seen from Earth. As the planet transits, gases in its atmosphere absorb a tiny fraction of the star’s light, leaving fingerprints specific to each gas. By splitting the star’s light into a rainbow of colors or spectrum, astronomers can look for those fingerprints. Kaltenegger and Traub studied whether those fingerprints would be detectable by JWST.
The transit technique is very challenging. If Earth were the size of a basketball, the atmosphere would be as thin as a sheet of paper, so the resulting signal is incredibly tiny. Moreover, this method only works when the planet is in front of its star, and each transit lasts for a few hours at most.
Kaltenegger and Traub first considered an Earth-like world orbiting a Sun-like star. To get a detectable signal from a single transit, the star and planet would have to be extremely close to Earth. The only Sun-like star close enough is Alpha Centauri A. No such world has been found yet, but technology is only now becoming capable of detecting Earth-sized worlds.
The study also considered planets orbiting red dwarf stars. Such stars, called type M, are the most abundant in the Milky Way – far more common than yellow, type G stars like the Sun. They are also cooler and dimmer than the Sun, as well as smaller, which makes finding an Earth-like planet transiting an M star easier.
An Earth-like world would have to orbit close to a red dwarf to be warm enough for liquid water. As a result, the planet would orbit more quickly and each transit would last a couple of hours to mere minutes. But it would undergo more transits in a given amount of time. Astronomers could improve their chances of detecting the atmosphere by adding the signal from several transits, making red dwarf stars appealing targets because of their more frequent transits.
An Earth-like world orbiting a star like the Sun would undergo a 10-hour transit once every year. Accumulating 100 hours of transit observations would take 10 years. In contrast, an Earth orbiting a mid-sized red dwarf star would undergo a one-hour transit once every 10 days. Accumulating 100 hours of transit observations would take less than three years.
“Nearby red dwarf stars offer the best possibility of detecting biomarkers in a transiting Earth’s atmosphere,” said Kaltenegger.
“Ultimately, direct imaging – studying photons of light from the planet itself – may prove a more powerful method of characterizing the atmosphere of Earth-like worlds than the transit technique,” said Traub.
Direct studies have already been used to create crude temperature maps of extremely hot, giant extrasolar planets. With next-generation instruments, astronomers may be able to study atmospheric compositions, not just temperatures. The characterization of a “pale blue dot” is the next step from there, whether by adding up hundreds of transits of one planet or by blocking out the starlight and analyzing the planet’s light directly.
In a best-case scenario, Alpha Centauri A may turn out to have a transiting Earth-like planet that no one has spotted yet. Then, astronomers would need only a handful of transits to decipher that planet’s atmosphere and possibly confirm the existence of the first twin Earth.
Source: Harvard Center For Astrophysics
Q & A with Dr. John Mather on the James Webb Space Telescope
The James Webb Space Telescope (JWST) is the much anticipated, long awaited “next generation” telescope. Planned for launch in 2013 October 2018, fall of 2021, JWST has been touted as the successor to the Hubble Space Telescope. With it, astronomers hope to look back in time to when the universe was just 200 million years old, and see the first stars and galaxies. The lead scientist guiding this project is Dr. John Mather, co-recipient of the 2006 Nobel Prize in Physics for his work with the Cosmic Background Explorer (COBE), which measured the black body form and anisotropy of the cosmic microwave background.
We were understandably honored when Dr. Mather contacted Universe Today, saying he’d like to talk with us about the status of JWST. “I figured it might be time to talk about what we’re doing,” he said, “because exciting things are starting to happen.”
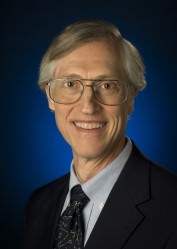
Universe Today: Dr. Mather, for over a decade we’ve been hearing about the Next Generation Space Telescope, which later was officially named the James Webb Space Telescope. Can you tell us how the concept for this telescope began?
John Mather: In 1989, even before the Hubble was launched, a conference was held about what the next space telescope should be. They discussed great telescopes of the future and from the proceedings, published a book. But they really didn’t consider that infrared was the great wave of the future. Then, in 1993, there was a committee called HST and Beyond. They published a lovely little report in 1996 that said there were two important things to do. One was to build an infrared telescope, unlike what the previous book had said, and the other was to build a telescope to search for Earth-like planets. At that point, astronomers were just recognizing that searching for extrasolar planets was possible. So in October 1995, NASA Headquarters called me up, gave me a list of scientists and engineers to contact and said to start planning. So we did, and we immediately came to a remarkable convergence of thought and opinion. We quickly agreed on a concept which met the wishes of the scientific community and came within the ambitions of NASA. You’ll find that the telescope we wanted to fly back then is very much like the one we’re going to fly in 2013.
UT: Can you give us an update on the status of the JWST right now?
Mather: The flight instrument hardware will be coming in from all over the world in the summer of 2010. The fine guidance sensor comes from Canada, one and a half instrument packages come from Europe and the rest comes from the US. So, in 18 months the instrument package starts going together, and then it meets up with the telescope around a year later. The four science instruments are a near-infrared camera, a near-infrared multi-object spectrograph, a mid-infrared instrument, and a tunable filter imager.
We just went through the Critical Design review for the instrument module. Last week we had hundreds of people come to look at everything and tell us if we’re doing it right. I think we passed, although I haven’t seen the official paperwork yet. But even I was impressed.
UT: The question many people ask me is, since Hubble has been so successful, why isn’t JWST going to be an optical telescope?
Mather: Why did the committee change from optical to infrared? It was twofold. One was that Hubble was getting so good, they could see it would be hard to beat it, no matter how big you built a telescope. Another thing happening was that people were seeing you could build big optical telescopes on the ground. The Keck Telescope was working really well, and people were starting to talk about adaptive optics, which meant even bigger telescopes on the ground were worthwhile. So those two things pointed us towards an infrared telescope. Also all the scientists of the JWST said we needed infrared. From the little capability we did have at the time, infrared was fascinating, finding that the most distant universe is exciting and is red-shifted from the visible. It starts out in ultraviolet and gets to infrared because of the great distances of these objects and the huge red shift they have. So if you want to do ultraviolet astronomy at the almost-edge of the universe you need an infrared telescope.
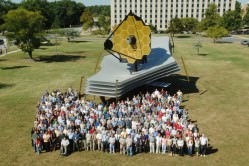
UT: Now that the infrared Spitzer Space Telescope is up and running so well, has that changed anyone’s mind, or does that make scientists want to go to the next level with infrared?
Mather: Yes, Spitzer has proved this is actually a fascinating territory. Spitzer is actually a little bitty telescope by modern standards; it’s only 3 feet wide, 85 cm. But it has been producing some astonishing surprises. They can see things out to very, very high red shifts, and none of those things were expected. So, that tells us infrared is where the wonderful discoveries will be. We now know we can do the technology, so let’s get a better telescope. The science is way, way exciting, and there’s so much out there waiting to be discovered.
UT: In your opinion, what will set the JWST apart from previous space telescopes?
Mather: Every telescope says, “I’m better than the one before me,” and we say the same. Of course, this telescope will see farther back in time with its infrared capability and its huge aperture; it will see through dust clouds to see where stars are being born; it will see things that are room temperature, like you and me, planets, or young stars being born. All those things can be seen directly with the infrared capability we have on this new telescope. Most of the work will be done in infrared, with some capability in the visible range.
But we’ve built a general purpose telescope. After launch, scientists can write proposals as they do for Hubble, for what they would like to observe, so they can observe whatever the hot topic is at that time.

UT: With your experience with COBE and the subsequent honors you received, how have you applied that to the JWST?
Mather: It wasn’t so much the honors that affected my life, it was the fact of having been through the process from the very beginning to the very end for a very radically designed observatory, which COBE was, that gave me the nerve to think big things. So when NASA Headquarters said they wanted a successor for Hubble, I thought that would be interesting, and I had enough nerve to say yes, I’d like to try that. COBE was very ambitious for the time, but small enough that I knew the engineers personally and I could talk to them any day about anything. So I thought I could graduate to a bigger project.
UT: And now you’re working with people from all around the world?
Mather: Yes, this is a huge deal. Our science team is about 19 people, from Europe, the US and Canada. The engineering team is over 2,000 people who are spread out all over the world. Clearly, I don’t know all of them. I work with the scientists most closely and talk with them about what we want to accomplish, and make sure we are accomplishing that. So I have a different role now. I don’t have hands-on responsibility for any hardware, but I work with the people who do. We have access to some of the very best people in the world on every topic.
UT: Can you talk about of the problems this telescope has had to overcome, the cost overruns and the delays it has had?
Mather: Number one, the cost overrun is not as big as is being portrayed by some folks that would like the money for their own project ideas. Originally Dan Goldin was the head of NASA when we started, and he said, “We want you to think of a way to do this observatory for a half a billion dollars in 1996 dollars.” We said we’d try. But we quickly realized building this was going to be hard. By the time we got ready to present it to the decadal survey in 2000, the cost was more like one billion dollars. Then, three years ago, we saw that the job was getting harder and we had to replan and rebudget. Now, if you count the entire NASA cost from beginning in 1995 to the end, somewhere after 2019 with inflation and civil servants (which we weren’t counting before) now it is roughly $4.5 billion in actual real dollars, not 1996 dollars. So there is cost growth, but we have had excellent success and we’re on track to launch this wonderful machine, which will be used by thousands of astronomers. And we haven’t had to change our plan or our total budget in three years, thanks to steady leadership from NASA HQ and brilliant technical work by the teams.
UT: That’s good to know. I think people have a general concept that the JWST has had a huge cost overrun.
Mather: Well, it’s not something small, and we wish we could have done better on that. But it’s about a factor of two growth, and not the factor of five that has been advertised by some people who should know better. This telescope will work for a long time. The requirement is five years, but we’re hoping to run it for ten. So, our project spans from 1995 to maybe 2024 when operations would end.
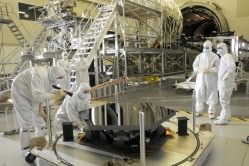
Let me give you some idea of what we needed to do to get ready and what we’ve been up to all this time. We developed a list of ten major technologies that we needed. The hardest thing was to develop the mirrors. That required twelve different contracts just for developing the competitors to where their designs were good enough, so that took quite a few years. The detectors clearly had to be improved over what we have on the Spitzer and Hubble telescopes. So now we have bigger and better detectors, and they are fabulous. One measure astronomers have is how many stray electrons do you get from the detectors. If you close off all the light you should get zero. We now have detectors that give off a few stray electrons per pixel per hour, which is almost perfect. It would be good to be even better, but this is fabulous. I’m impressed.
We needed to improve on the refrigerators in space. We started off saying we need to get a radiatively cooled telescope so that it would be cool enough by itself, and that’s mostly true. But it turns out we still need an active refrigerator to keep the longest wavelength detectors cold, so we had to develop that.
So, those are just some of the things we had to design, and all the technology development was finally finished in 2007 and passed the review board’s approval, who said,”Yes, those things are finally ready now to be built.”
So, just getting to 2007 was a long time, and I don’t think people have really appreciated what it takes to get new technologies ready. On the other hand, we’ve been blessed by not having to “back up.” We put enough planning and effort into these technologies that they work now. That was one of the things we learned from the Hubble project, which was, don’t finish your design until you know what you’re supposed to build.
UT: How about your testing process. Is it pretty rigorous?
Mather: That is another lesson we had to learn from Hubble. If you don’t test it, it’s not going to work. We’ve learned to have a very determined and rigorous process. They did enough testing on the Hubble that they could have known about the mirror focus problems. The mirror manufacturer had two tests that didn’t agree and they decided to ignore one of them instead of tracking down the reason, and that turned out to be foolish and expensive.
We have a generalization that if anything really matters, do it twice. We will actually test the telescope cold in the big vacuum tank down at Johnson Space Center. So it will be a full-scale end-to-end “light-in-at-the-beginning, light-out-at-the end” sort of test, something they could not do for Hubble. But they knew they could go fix Hubble in space, and we know we can’t fix JWST, since the telescope will be at the L-2 point, about 1.5 million kilometers away from Earth, which is about four times further away from the Earth than the Moon.
This is a complicated project, but our approach for doing a complicated project is dramatically different from when I was a young feller. When I got here to Goddard, we used pencils and slide rules, and computers were pretty new and most people didn’t have them. Now we have computers everywhere that keep track of our documents. We can do systems engineering, and even can do very accurate, complete simulations to know if something will fit together and work before we even built it. So the world has changed, and it’s a wonderful thing to see. So this is why we are now able to build this observatory for about the same real cost as it took to get the Hubble launched and working. But JWST is so much bigger and more powerful.
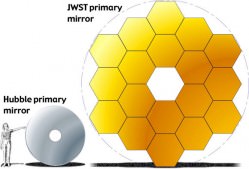
UT: Can you tell us about the design for the mirror for the JWST?
Mather: The hardest thing to build was the mirror, because we needed something that is way bigger than Hubble. But you can’t possibly lift something that big or fit it into a rocket, so you need something that is lighter weight but nonetheless larger, so it has to have the ability to fold up.
The mirror is made of light-weight beryllium, and has 18 hexagonal segments. The telescope folds up like a butterfly in its chrysalis and will have to completely undo it self. It’s a rather elaborate process that will take many hours. The telescope is huge, at 6.5 meters (21 feet), so it’s pretty impressive.
The sun shield is completely new, and it too will have to deploy. So, what was wrapped up into a small cylinder, relatively speaking becomes a giant shield about as big as a tennis court. It’s huge. All this happens in multiple stages and will take days. We hired a company, Northrop Grumman that had experience unfolding things in space, and they tell us this is definitely not the most complicated thing they’ve unfolded in space, which is reassuring.
Video of the JWST deploying in space:
UT: Has there been any discussion of first light and what the JWST will look at first?
Mather: Yes, a little. That will be the fun part after we get the thing put together.
UT: Do you have any favorite suggestions?
Mather: I think we should start with easy targets that will be pretty, that will enable the public to say, “Oh, I see its working!” Some of the first observations can be done when we’re setting up the telescope, even before it’s fully adjusted. Because it is deployed after launch and the mirror isn’t close to the right shape at first, we’ll be working up to this gradually. There’s a test model at Ball Aerospace in Boulder Colorado, where we get to practice putting the 18 mirror segments into position. Each segment has 7 motors on it to control the position and curvature, so we have to rehearse this one.
This is something they couldn’t do with Hubble. They wished they could, and it did have motors but they couldn’t push hard enough. That’s an interesting story. We learned from Hubble how to correct the optics based on the images we were getting, so we’re doing it on purpose for this telescope.
UT: There has been some controversy about how the JWST will be launched.
Mather: We’ll take the telescope to French Guiana and load it in the rocket down there. The ESA is buying the launch vehicle for us; it’s the Ariane 5 rocket, a commercial product from Europe and they’ve had a good run lately, so it’s very reliable.
Naturally that caused a lot of controversy. Even if Europe was giving us the launch vehicle, so to speak, there were people here who did not want to accept it. It took headquarters two years to accept it. That cost us money. The only reason it was accepted was that we got a new administrator who would accept it. That was Mike Griffin, so I want to say, “thank you very much Mike Griffin!”
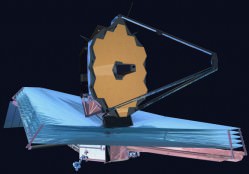
UT: Your team still has a lot to do before 2013, which will probably be here before you know it!
Mather: Yes, I know. It’s been over 13 years now since NASA contacted me about this, but now the end is coming up fast. We have plenty of technical challenges ahead of us in putting everything together. And we haven’t gotten far enough to along yet find out how many things we broke or how many mistakes we made, but I think we’re pretty good at figuring them out before we make them.
It’s going to be very exciting to put the equipment together for the first time. We’ve got the pieces, we’ve got the picture on the box to show where they go, and pretty soon we get to prove that they work together, or not. By the time we receive all the parts here at Goddard, they will all have been tested individually, so they’re supposed to play together just fine. But nature doesn’t like arrogance, so we have to test the whole thing from beginning to end, just as we’re going to use it in flight. After we put it together here, we take it down to Johnson Spaceflight Center, and put it in the giant vacuum tank there. That will be an extraordinary process.
UT: Thank you so much for talking with us.
Mather: This has been fun. I love telling my story and I’m glad you want to tell it with us. I figured it might be time to talk about what we’re doing because exciting things are starting to happen. Magnificent things are happening. We’ve got the Kepler Observatory up now, and hopefully they’ll find a handful of Earth-like planets to track down and we’ll take a closer look at them.
Could the First Stars Have Been Powered by Dark Matter?
Early stars that began to form about 200 million years after the Big Bang were strange creatures. From observation, the earliest stars (formed from coalescing primordial gas clouds) were not dense enough to support fusion reactions in their cores. Something within the young suns was counteracting the collapsing gas clouds, preventing the core reactions from taking place. Yet, they still produced light, even in absence of nuclear processes. Could dark matter have had a part to play, fueling the stellar bodies and sparking early stars to life?
New research indicates that the energy generated by annihilating dark matter in the early universe may have powered the first stars. How? Well, the violent early universe will have had high concentrations of dark matter. Dark matter has the ability to annihilate when it comes into contact with other dark matter matter, it does not require anti-dark matter to annihilate. When “normal” matter collides with its anti-component (i.e. electron colliding with positron), annihilation occurs. Annihilation is a term often used to describe the energetic destruction of something. While this is true, the annihilation products from dark matter include huge amounts of energy to create neutrinos and “ordinary matter” such as protons, electrons and positrons. Dark matter annihilation energy therefore has the ability to condense and create the matter we see in the Universe today.
“Dark matter particles are their own anti. When they meet, one-third of the energy goes into neutrinos, which escape, one-third goes into photons and the last third goes into electrons and positrons.” – Katherine Freese, Theoretical Physicist, University of Michigan.
Katherine Freese (University of Michigan), Douglas Spolyar (University of California, Santa Cruz) and Paolo Gondolo (University of Utah in Salt Lake City) believe the strange physics of the early “dark stars” may be attributed to dark matter. For a star to form from stellar gas cloud to a viable, burning star, it must cool first. This cooling allows the star to collapse so the gas is dense enough to kick-start nuclear reactions in the core. However, early stars appear to have some form of energy acting against the cooling and collapse of early stars, fusion shouldn’t be possible, and yet the stars still shine.
The group believe that early stars may have passed through two stages of development. As the gas clouds collapse, the stars go through a “dark matter phase”, generating energy and producing normal matter. As the phase progresses, dark matter will slowly be used up and converted into matter. As the star becomes sufficiently dense with matter, fusion processes take over, starting the “fusion phase”. Fusion in turn generates heavier elements (such as metals, oxygen, carbon and nitrogen) during the lifetime of the star. When the early stars’ fuel is used up, it will go supernova, exploding and distributing these heavy elements throughout space to form other stars. The “dark matter phase” appears only to have existed in the very first stars (a.k.a. “population three stars”); later stars are supported by fusion processes only.
However, this exciting new theory will have to wait until the James Webb Telescope goes into operation in 2013 before population three stars can be observed with any great accuracy. Light may then be shone on the processes powering the first “dark stars” of our early Universe.
Source: Physorg.com
Grapple Attachment May Be Added to the James Webb Telescope
When the James Webb telescope launches in 2013, it’ll be the most powerful telescope ever deployed in space. To the get the best view, using the least fuel, it’ll sit in a stable orbit about 1.6 million km (1 million miles) from Earth. Unlike Hubble, it was never meant to be repaired. But NASA announced that it’s considering installing a grapple attachment anyway, just to be safe.
Since the new Orion spacecraft will be capable of taking astronauts to the Moon and back, NASA is investigating how feasible it’ll be to send astronauts to the James Webb telescope to perform emergency servicing operations. Although the observatory is meant to never be serviced, it’s good to keep your options open.
Engineers are currently working out what would be the best kind of hardware they could add to the telescope, so that future astronauts or robotic missions could link up with the telescope and perform repairs.
Original Source: NASA News Release