Everyone knows just how fun magnets can be. As a child, who among us didn’t love to see if we could make our silverware stick together? And how about those little magnetic rocks that we could arrange to form just about any shape because they stuck together? Well, magnetism is not just an endless source of fun or good for scientific experiments; it’s also one of basic physical laws upon which the universe is based.
The attraction known as magnetism occurs when a magnetic field is present, which is a field of force produced by a magnetic object or particle. It can also be produced by a changing electric field and is detected by the force it exerts on other magnetic materials. Hence why the area of study dealing with magnets is known as electromagnetism.
Definition:
Magnetic fields can be defined in a number of ways, depending on the context. However, in general terms, it is an invisible field that exerts magnetic force on substances which are sensitive to magnetism. Magnets also exert forces and torques on each other through the magnetic fields they create.
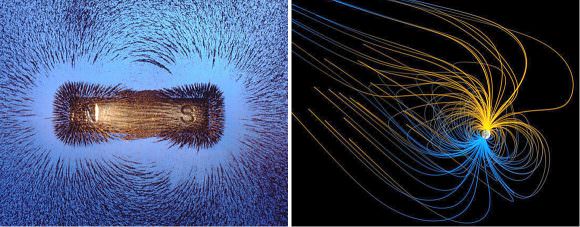
They can be generated within the vicinity of a magnet, by an electric current, or a changing electrical field. They are dipolar in nature, which means that they have both a north and south magnetic pole. The Standard International (SI) unit used to measure magnetic fields is the Tesla, while smaller magnetic fields are measured in terms of Gauss (1 Tesla = 10,000 Guass).
Mathematically, a magnetic field is defined in terms of the amount of force it exerted on a moving charge. The measurement of this force is consistent with the Lorentz Force Law, which can be expressed as F= qvB, where F is the magnetic force, q is the charge, v is the velocity, and the magnetic field is B. This relationship is a vector product, where F is perpendicular (->) to all other values.
Field Lines:
Magnetic fields may be represented by continuous lines of force (or magnetic flux) that emerge from north-seeking magnetic poles and enter south-seeking poles. The density of the lines indicate the magnitude of the field, being more concentrated at the poles (where the field is strong) and fanning out and weakening the farther they get from the poles.
A uniform magnetic field is represented by equally-spaced, parallel straight lines. These lines are continuous, forming closed loops that run from north to south, and looping around again. The direction of the magnetic field at any point is parallel to the direction of nearby field lines, and the local density of field lines can be made proportional to its strength.
Magnetic field lines resemble a fluid flow, in that they are streamlined and continuous, and more (or fewer lines) appear depending on how closely a field is observed. Field lines are useful as a representation of magnetic fields, allowing for many laws of magnetism (and electromagnetism) to be simplified and expressed in mathematical terms.
History of Study:
The study of magnetic fields began in 1269 when French scholar Petrus Peregrinus de Maricourt mapped out the magnetic field of a spherical magnet using iron needles. The places where these lines crossed he named “poles” (in reference to Earth’s poles), which he would go on to claim that all magnets possessed.
During the 16th century, English physicist and natural philosopher William Gilbert of Colchester replicated Peregrinus’ experiment. In 1600, he published his findings in a treaties (De Magnete) in which he stated that the Earth is a magnet. His work was intrinsic to establishing magnetism as a science.
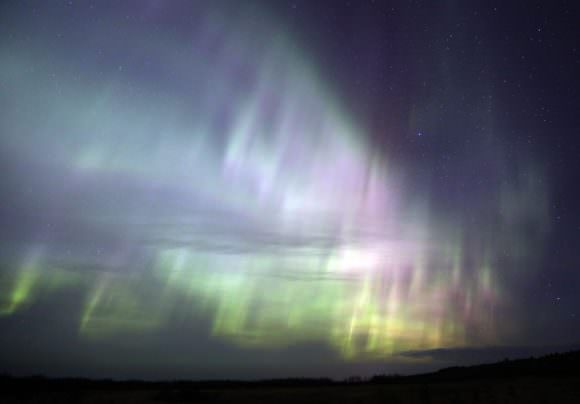
In 1750, English clergyman and philosopher John Michell stated that magnetic poles attract and repel each other. The force with which they do this, he observed, is inversely proportional to the square of the distance, otherwise known as the inverse square law.
In 1785, French physicist Charles-Augustin de Coulomb experimentally verified Earths’ magnetic field. This was followed by 19th century French mathematician and geometer Simeon Denis Poisson created the first model of the magnetic field, which he presented in 1824.
By the 19th century, further revelations refined and challenged previously-held notions. For example, in 1819, Danish physicist and chemist Hans Christian Orsted discovered that an electric current creates a magnetic field around it. In 1825, André-Marie Ampère proposed a model of magnetism where this force was due to perpetually flowing loops of current, instead of the dipoles of magnetic charge.
In 1831, English scientist Michael Faraday showed that a changing magnetic field generates an encircling electric field. In effect, he discovered electromagnetic induction, which was characterized by Faraday’s law of induction (aka. Faraday’s Law).
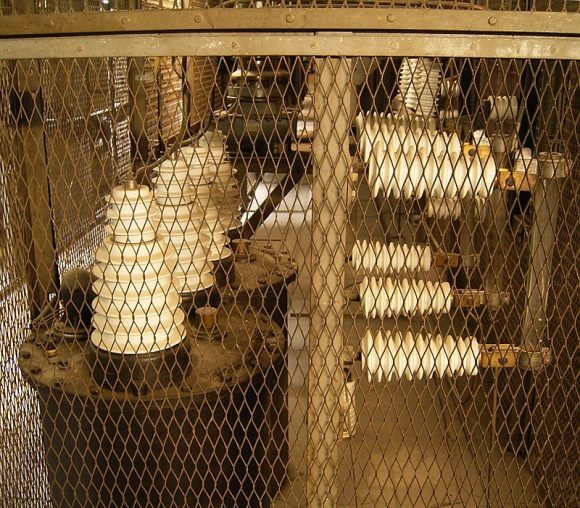
Between 1861 and 1865, Scottish scientist James Clerk Maxwell published his theories on electricity and magnetism – known as the Maxwell’s Equations. These equations not only pointed to the interrelationship between electricity and magnetism, but showed how light itself is an electromagnetic wave.
The field of electrodynamics was extended further during the late 19th and 20th centuries. For instance, Albert Einstein (who proposed the Law of Special Relativity in 1905), showed that electric and magnetic fields are part of the same phenomena viewed from different reference frames. The emergence of quantum mechanics also led to the development of quantum electrodynamics (QED).
Examples:
A classic example of a magnetic field is the field created by an iron magnet. As previously mentioned, the magnetic field can be illustrated by surrounding it with iron filings, which will be attracted to its field lines and form in a looping formation around the poles.
Larger examples of magnetic fields include the Earth’s magnetic field, which resembles the field produced by a simple bar magnet. This field is believed to be the result of movement in the Earth’s core, which is divided between a solid inner core and molten outer core which rotates in the opposite direction of Earth. This creates a dynamo effect, which is believed to power Earth’s magnetic field (aka. magnetosphere).
Other celestial bodies have been shown to have magnetic fields of their own. This includes the gas and ice giants of the Solar System – Jupiter, Saturn, Uranus and Neptune. Jupiter’s magnetic field is 14 times as powerful as that of Earth, making it the strongest magnetic field of any planetary body. Jupiter’s moon Ganymede also has a magnetic field, and is the only moon in the Solar System known to have one.
Mars is believed to have once had a magnetic field similar to Earth’s, which was also the result of a dynamo effect in its interior. However, due to either a massive collision, or rapid cooling in its interior, Mars lost its magnetic field billions of years ago. It is because of this that Mars is believed to have lost most of its atmosphere, and the ability to maintain liquid water on its surface.
When it comes down to it, electromagnetism is a fundamental part of our Universe, right up there with nuclear forces and gravity. Understanding how it works, and where magnetic fields occur, is not only key to understanding how the Universe came to be, but may also help us to find life beyond Earth someday.
We have written many articles about the magnetic field for Universe Today. Here’s What is Earth’s Magnetic Field, Is Earth’s Magnetic Field Ready to Flip?, How Do Magnets Work?, Mapping The Milky Way’s Magnetic Fields – The Faraday Sky, Magnetic Fields in Spiral Galaxies – Explained at Last?, Astronomy Without A Telescope – Cosmic Magnetic Fields.
If you’d like more info on Earth’s magnetic field, check out NASA’s Solar System Exploration Guide on Earth. And here’s a link to NASA’s Earth Observatory.
We’ve also recorded an episode of Astronomy Cast all about planet Earth. Listen here, Episode 51: Earth.
Sources:
I have always wondered what mechanism does a magnetic force uses to move objects attracted to a magnet or being repelled as in same magnetic poles. This mechanism is invisible, and works through non-metallic barriers such as paper, wood etc.
In a similar situation with the case of forces of gravity, we now know that gravity works by distorting space-time, so objects within it is sort of pushed around by the thinned-out space. How does magnetism do it?
Physicists say that it is the exchange of virtual photons. The idea is that all forces are manifest by the exchange of the characteristic force particles. In the case of electromagnetism (EM), it’s photons. When an EM field is being measured, you are actually measuring the force exerted by these virtual photons being exchanged between the source of the field and the object you’re holding onto. The term “virtual” is used insofar as you can’t see them, but physicists are convinced that they are there. The math works out, as I understand it.
The reason why most metallic objects provide effective shielding against EM fields is due to the electrical conductivity of the material. The virtual photons are absorbed/conducted-away/something like that.
I have difficulty with the concept of the concept of exchanging virtual force particles, but that’s the standard explanation.
I see you are slowly realizing the importance of electromagnetism in the structure and function of the universe. The massive magnetic fields being measured by nearly every NASA mission to the sun and deep space are undeniable. The obvious fact that accompanies these findings is that there is an electric current (flow of charged particles) that occurs concomitantly with every magnetic field.
It is clear that everything we see in space is due to the radiation generated from plasma in the glow mode. So it would be wise to consider the electromagnetic nature of the universe rather than create mystical entities that defy the laws of physics to explain it. No matter what mathematicians propose, their lack of observation and experimental confirmation relegates their concepts to the science fiction realm….not one of scientific fact.
Your preoccupation with gravity will eventually fade as you begin to comprehend the massive electromagnetic forces responsible for star and galaxy formation. Once the obvious predominance of plasma physics and electrical engineering principles becomes clear to you there will be no further need for gravity based inventions such as dark matter and black holes.
It is with a sigh of relief that I finally see papers being published in mainstream journals that suggest dark matter may not exist at all (Astrophysics Journal). Recognizing the spin of galaxies, stars and planets could not possibly be accounted for by dark matter or gravity is just a first step. Only an electromagnetic model simply and elegantly accounts for these and all other findings being made today.
I would humbly suggest that you view Dr. Scott’s website (electric-cosmos.org) and see the Voyager I findings that confirm the electric sun model. This was proposed in great detail by Ralph Juergens in the 70’s and all recent data confirms the model. It is beyond debate that the “photospheric granules” are actually anode tufts composed of plasma in the glow mode. Dr. Scott”s recent presentation explaining the exact nature of the two forms of “solar wind” is totally consistent with this model as well as all other recent evidence. His knowledge is beyond reproach having written the major textbook used in the U.S. for graduate electrical engineering programs. He also uses profound common sense. For example he chuckles when referring to the massive solar emissions being photographed today and their description by NASA astrophysicists as magnetic fields of the sun. He reminds us that magnetic fields cannot be visualized and explains the exact nature of the visual findings.
My hope is that you will visit Dr. Scott’s site and view this material with an open mind. With your intelligence and enthusiasm you can make a tremendous contribution towards a true understanding of the “Universe Today”.
“Slowly realizing”? There’s hardly any realization here, the importance of electromagnetism in the Universe – as a fundamental force of nature – is well known to us here. As it is well known to any science website.
@btraymd…??? What are you talking about? I’m not sure you’re paying attention.
You have a very strange sense of what the Universe is and how things work (to the best of modern knowledge)…and your dismissal does not improve your case. In fact, the example about “chuckling” at NASA for a glib, public-oriented picture caption is absurd.
NASA knows that the bright arcs of light sticking out from the Sun are not “magnetic fields” being observed directly. The simplistic language that you might be referring to is for publicly-released picture captions. And even so, I have been noticing that NASA and others have begun to spell out that the light is from the electron transitions of the ions that travel along the “field lines”. Thus, the stronger regions of the emerging magnetic field is illuminated by the concentration of the ions. Physicists, including those at NASA, have known that for a long time. It’s just the photoelectric effect. However, different people have different expectations for scientific literacy among the public at large.
As far as gravity goes, it’s obviously there, and it’s not clearly linked to EM, other than from what General Relativity suggests (correctly predicts the photon path deflection from a given frame of reference around large bodies, etc).
Interesting article. Apart for the fun stuff. I noticed a picture in the middle of the article of an electrical grid point of distribution that was enclosed in a Faraday cage. Why was that done? For a solar storm or an EMP from a nuclear detonation in the atmosphere? Curious , but I thought a good Faraday cage had to be solid and fairly substantial. Is there a trick here I am missing? Would that chicken wire cage stop anything of any significant energy?
I’m not sure why they are necessary in the above picture, but I can tell you that Faraday cages are designed so that you block EM radiation that you don’t want (typically RF or microwave), but allow EM radiation that you do want (light).
It is a function of the size of the holes. The perforated metal plate in the window of microwave ovens is a Faraday structure, where the holes are significantly smaller than the extinction ratio of the generated microwaves. In other words, the microwaves generated in the oven do not “see” the holes, but are stopped as if that perforated sheet were solid.
By contrast, with wavelengths on the order of 100s of nanometers, light passes through just fine, so you can see the food without getting baked.
Back to the picture, it looks like it could be just a protective cage to prevent people and animals from coming into contact with the equipment within. Holes that large are only going to block RF, possibly nothing more than any EM field of about 60Hz, I would guess.
What causes the ripples and swirls in the aurora curtains? Why do they change so rapidly?
“What is a Magnetic Field?”
Your answer:
“… an invisible field that exerts magnetic force”
seriously? that’s the best you could come up with?
not good enough.
Then perhaps you should read past the first paragraph of the definition section. If that’s still not good enough for you, you can always write your own articles.