Time is relative, as they say, particularly for mid-day meals. As special relativity shows, the measure of any two clocks depends on their motion relative to each other. The greater their relative speed, the slower each clock is relative to each other. So, since we see distant galaxies speeding away from us, we should also see time move more slowly. Right?
While that is true, we should be careful not to confuse what is going on. One of the central ideas to note is that each observer will experience time at the standard rate. Look at your watch while speeding along in a rocket, and the seconds will tick away just as they always do. The same is true for an observer on Earth. It’s only when an observer on Earth and on a speeding rocket compare their watches that things get confusing. Each observer thinks the other has a slow watch.
The other thing to keep in mind is that special relativity doesn’t apply globally when things like gravity and dark energy come into play. For that, you need general relativity, but the results are much the same. In the case of distant galaxies, they aren’t speeding away from us through space like a rocket. Instead, space itself is expanding between us and those galaxies. Because of this cosmic expansion, the light from them is shifted to the red, hence their high redshift. This cosmic expansion also stretches the apparent time between ticks and tocks, making the rate of time in those galaxies appear slower to us.
Because of this, one of the predictions of cosmology is that when we look at distant objects, peering into the deep and past universe, time should appear to move more slowly. From our vantage point, time was slower in the early universe. Much slower.
Strange as this is, we have evidence to support it. When we look at distant supernovae, we see a time dilation effect. Type Ia supernovae are used as standard candles because they have a consistent maximum brightness, but they also have a consistent light curve, meaning that they all brighten and fade over a similar period of time. Astronomers have shown that the more distant a Type Ia supernova, the more stretched out its light curve is. In other words, it takes longer for a distant supernova to brighten and fade than a closer supernova. Time appears to slow down with distance.
According to the standard cosmological model, this correlation between distance and time dilation should hold all the way back to the cosmic microwave background. And while all the evidence we’ve gathered supports that theory, our evidence has been somewhat limited. The most distant supernovae we’ve observed come from a time when the universe is a bit less than 4 billion years old. But a new study has proven the model for even greater distances, thanks to quasars.
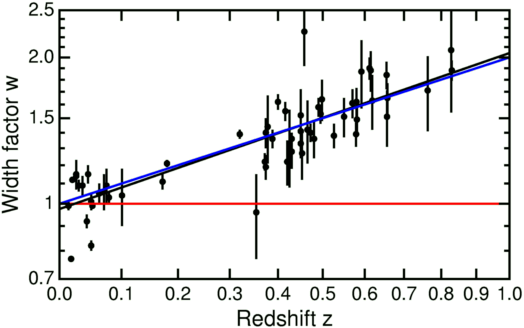
Quasars are tremendously powerful active galactic nuclei (AGNs) powered by supermassive black holes in early galaxies. They are called quasars or quasi-stellar objects because they were first discovered as bright points of radio light. Quasars don’t emit light in a single burst like supernovae, but they do have a kind of timing clock to them. Because of the finite speed of light, it takes a bit of time for effects to travel across the span of an AGN. Because of this, fluctuations in the intensity of a quasar depend on the size of the AGN. So quasars of the same size fluctuate in intensity at the same rate.
Before this recent study, there wasn’t a way to distinguish whether slower fluctuating quasars were an effect of time dilation, or whether they were simply larger AGNs. So the team looked at 190 distant quasars at a range of wavelengths. They combined 20 years of data gathered at green, red, and infrared wavelengths and were able to create a standard measure of the tick-and-tock of quasar fluctuations. When they looked at the quasars by distance, statistically there was a correlation. The more distant the quasar, on average the slower its clock.
The study was able to extend time dilation observations all the way back to when the universe was just a billion years old. From our perspective, a second from that epoch appears to last five seconds. This agrees with general relativity and the standard cosmological model.
While not unexpected, the result is yet another wonderful way to show us that we understand cosmic evolution. Space really is expanding, powered by dark energy, and the universe really did begin with a big bang, where time appeared to move much more slowly than it does now.
Reference: Goldhaber, Gerson, et al. “Timescale stretch parameterization of type Ia supernova B-band light curves.” The Astrophysical Journal 558.1 (2001): 359.
Reference: Lewis, Geraint F. and Brewer, Brendon J. “Detection of the cosmological time dilation of high-redshift quasars.” Nature Astronomy (2023).
Yes, well, a little digging showed me that Friedmann’s FLRW solutions included a stationary (“Einstein’s cylindrical”) universe where vacuum has a gravitational Hansen potential, with LCDM observing flat space a dead ringer for the simplest choice.
And with later advances in effective quantum field theory (e.g. Donohue’s straightforward quantization of GR) the gravitational potential of free space one can ask why not make these theories approximations of each other – GR is much better to work out how the universe reacts, EFT is more basic and better at microscale- since that would work out to preserve energy, probabilities and through the potential give the vaunted lacking energy localization of approximative GR?
So maybe it is actually the non-reciprocal GR effect of gravitational time dilation we look at, somewhat like the familiar reciprocal time dilation of special relativity, and space expansion is an odd interpretation from a time when curved space meant the universe could have any geometry.