The theory of general relativity is packed with strange predictions about how space and time are affected by massive bodies. Everything from gravitational waves to the lensing of light by dark matter. But one of the oddest predictions is an effect known as frame-dragging. The effect is so subtle it was first measured just a decade ago. Now astronomers have measured the effect around a white dwarf, and it tells us how some supernovae occur.
In general relativity, gravity is not a force. The presence of a mass bends space around it, and this means that objects moving near the mass are deflected from a straight path. This deflection looks as if the object is being pulled toward the mass as if by a force we call gravity. When a large mass is rotating, space also twists slightly in the direction of rotation. It is this effect that is known as frame-dragging.
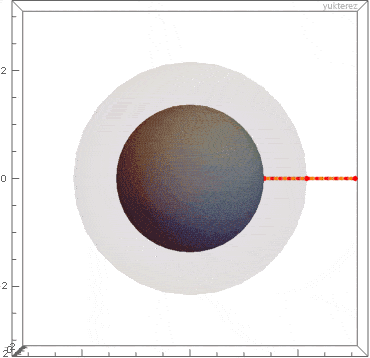
You can see an illustration of frame-dragging in the figure above. The central object is a massive rotating body, such as a black hole. The red dots represent points that are “at rest,” which means they aren’t moving through space. Instead, they move because space around the body is twisting due to the rotation. This frame-dragging effect is in addition to any orbital motion an object might have, and it is part of the reason why the accretion disk around a black hole can get so extremely hot.
Near Earth, the frame-dragging effect is very small. So small that it took a special satellite to measure it. Known as Gravity Probe B, the spacecraft contained one of the most spherical objects ever made. Once in space, the sphere was set spinning and watched over time.
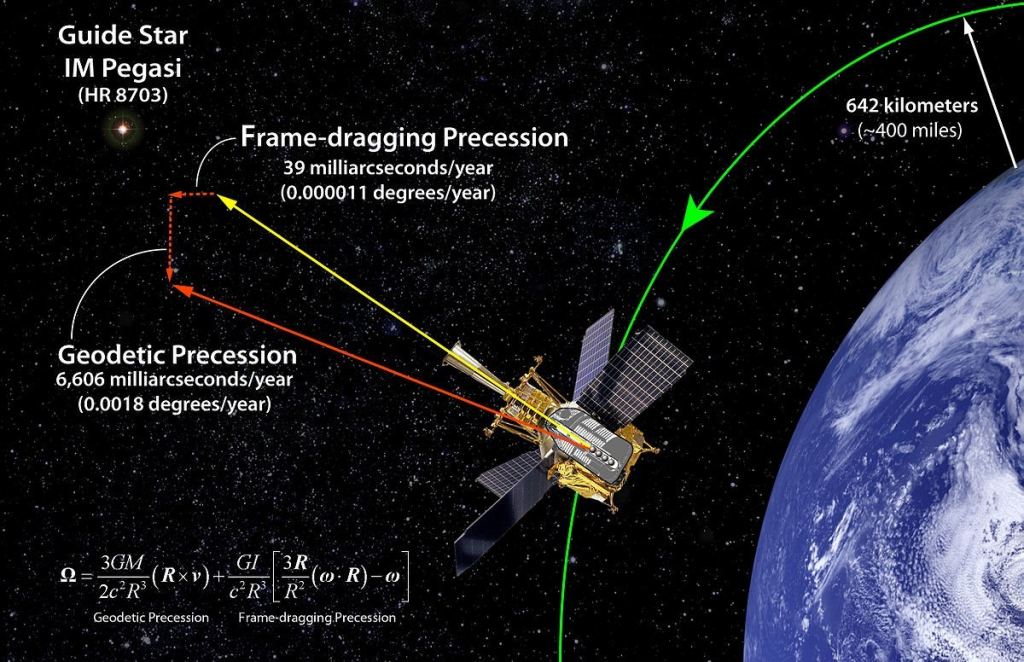
Without frame-dragging, a spinning sphere orbiting the Earth should always keep the same orientation, like a gyroscope. Earth’s gravity can’t cause it to twist on its own. But frame-dragging can. Because of Earth’s rotation, the region of space closer to the Earth twists just slightly faster than the region of space farther away. This means the part of the sphere that’s closer to Earth gets a little push, and as a result, it’s orientation changes over time. We call this Lense–Thirring precession. In 2015 the team measured this precession, and it agreed perfectly with general relativity.
While the frame-dragging effect is larger around massive bodies like white dwarfs and neutron stars, it isn’t easy to measure. To measure the frame-dragging of a body you need to have something orbiting it. Luckily for us, many white dwarfs and neutron stars are part of a binary system. So recently a team used a binary system to study frame dragging.
In 1999, the Australian Parkes Radio Telescope discovered the pulsar PSR J1141-6545. It is a neutron star that’s in a binary orbit with a white dwarf star. The distance between these two stars is only about the width of the Sun, and they orbit each other every five hours.
Because pulsars emit a sharp radio pulse at regular intervals, astronomers can use them to make extremely accurate measurements of the pulsar’s motion and orbit. The measurements are so precise that we can use them to measure the effects of general relativity, including frame dragging. Because the white dwarf is rotating, the orbit of the pulsar precesses slightly over time. The amount of precession depends on the mass and rotational speed of the white dwarf.
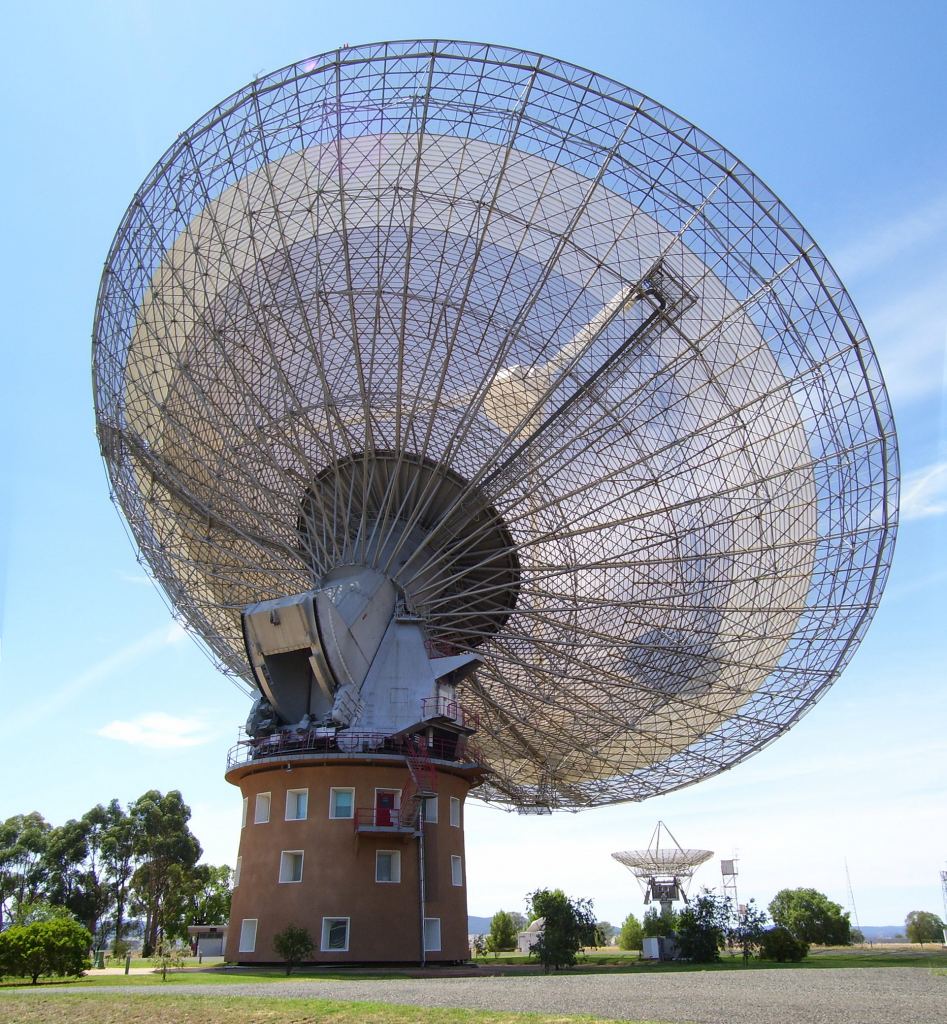
After observing the pulsar for twenty years, the team not only observed frame-dragging, they used it to measure the rotational speed of the white dwarf. They found that it rotates once every 100 seconds, which is quite fast for a white dwarf.
The results agree with a popular model about how close binary systems evolve. Pulsars form when large stars die and become supernovae. This means the binary system was once a binary system where a large star orbited the white dwarf. As the star reached the end of its life, material from its outer layer would have been captured by the white dwarf, causing it to spin faster. The observations show that the white dwarf formed before the pulsar.
All this from an amazing work of astronomy, measuring relativistic frame-dragging in a star 12,000 light-years away.
Reference: Everitt, C. W. F., et al. “The Gravity Probe B test of general relativity.“
Reference: V. Venkatraman Krishnan, et al. “Lense–Thirring frame dragging induced by a fast-rotating white dwarf in a binary pulsar system“