Earth observations are one of the most essential functions of our current fleet of satellites. Typically, each satellite specializes in one kind of remote sensing – monitoring ocean levels, for example, or watching clouds develop and move. That is primarily due to the constraints of their sensors – particularly the radar. However, a new kind of sensor undergoing development could change the game in remote Earth sensing, and it recently received a NASA Institute for Advanced Concepts (NIAC) grant to further its development.
Continue reading “How a Single Atomic Sensor Can Help Track Earth’s Glaciers”When Light Just Isn’t Fast Enough
Take a speed of light trip across the solar system starting at the Sun
We’ve heard it over and over. There’s nothing faster than the speed of light. Einstein set the speed limit at 186,000 miles per second (299,792 km/sec). No material object can theoretically travel faster. For all practical purposes, only light is lithe enough to travel at the speed of light.
Moving in such haste, a beam of light can zip around the Earth 8 times in just one second. A trip to the moon takes just 1.3 seconds. Fast for sure but unfortunately not fast enough. Hit play on the video and you’ll soon know what I mean. The view begins at the Sun and travels outward into the solar system at the speed of light.
Planet Distance in AU Travel time .................................................................... Mercury 0.387 193.0 seconds or 3.2 minutes Venus 0.723 360.0 seconds or 6.0 minutes Earth 1.000 499.0 seconds or 8.3 minutes Mars 1.523 759.9 seconds or 12.6 minutes Jupiter 5.203 2595.0 seconds or 43.2 minutes Saturn 9.538 4759.0 seconds or 79.3 minutes Uranus 19.819 9575.0 seconds or 159.6 minutes Neptune 30.058 14998.0 seconds or 4.1 hours Pluto 39.44 19680.0 seconds or 5.5 hours ...................................................................
Distances and light times to the planets and Pluto (from Alphonse Swinehart)
You might first think that moving that fast will get us across the orbits of the eight planets in a hurry. I shouldn’t have been surprised, but I found myself already getting impatient by the time Mercury flew by … after 3.2 minutes. Earth was still 5 minutes away and Jupiter another 40! That’s why the video cuts off at Jupiter – no one would stick around for Pluto’s appearance 5 1/2 hours later.
As the video tediously but effectively demonstrates we live in a solar system where a few planets are separated by vast spaces. Not even light is fast enough to satisfy the human need for speed. But just to put things in perspective, the fastest current human-made objects is NASA’s Voyager I spacecraft, which recently reached interstellar space traveling at 38,000 mph (17 km/sec) or nearly 18,000 times slower than light speed.
Let’s explore further. Any material object, a Skittle for instance, moving that fast would become infinitely massive. Why? You’d need an infinite amount of energy to accelerate the Skittle to the exact speed of light. Since matter and energy are two faces of the same coin, all that energy creates an infinitely massive Skittle. Sweet revenge if there ever was.
You can however accelerate the pill-like candy to 99.9999% light speed with a finite if incredibly large amount of energy. Einstein’s cool with that. Here’s the weird thing. If you were travelling along at the speed of light it would look like a perfectly normal piece of candy, but if you were to look at it from the outside world, the sugary treat would be the entire universe. Both viewpoints are equally valid, and that’s the essence of relatively.
Wave-particle duality of light
To better imagine a day in the life of a photon, let’s go along for the ride. Photons are the particle form of light, which for a long time was only understood as waves of electromagnetic energy. In the weirdness of quantum world, light is both a particle and a wave. From our perspective, a photon rip by at 186,000 miles per second, but to the photon itself, the world stands still and time stops. Photons are everywhere at once. Omnipresent. No time passes for them.
In relativity theory, the movement of anything is defined entirely from an observer’s point of view. From the photon’s perspective, it’s at rest. From ours, it’s moving across time and space. We all have our own “coordinate frame”, so that wherever we are, we’re at rest. That’s relativity for you – all frames are equally valid.
Let say you’re in a plane. That sad bag of pretzels you were just handed is at rest because it’s in your coordinate frame. The person next to you is likewise at rest (and hopefully not snoring). Even the plane’s at rest. According to Einstein, it’s just as valid to picture the world outside the airplane window moving while the plane itself remains at rest. Next time you fly, close your eyes once the plane reaches altitude and a constant speed. You’ll hear the noise of engines, but there’s no way to know you’re actually moving.
Relativity also predicts that objects contract in the direction of their motion. Strange as it sounds, this has been verified by many experiments. The faster things travel, the more they contract.
The effect doesn’t become noticeable until an object approaches light speed, but the Apollo 10 service and crew modules reached a velocity of 0.0037% the speed of light. From the perspective of someone on the ground, the 11.03-meter-long module shrank by approximately 7.5 nanometers, an exceedingly tiny but measurable amount. (A sheet of paper is 100,000 nanometers thick). Likewise, distances contract, bottoming out at zero at light speed.
Length contraction occurs because a stationary observer sees a speedy spaceship traveler’s time tick by more slowly. Since light is measured in time units – light seconds, light years – in order for the two to agree on the speed of light (a constant across the universe) the traveler’s “ruler” has to be shorter. And it really is from your stationary perspective if you could somehow peer inside the ship. Traveling at 10% light speed, a 200-foot spaceship shrinks to 199 feet. At 86.5%, it’s 100 feet or half the size and at 99.99% only 3 feet!
We’ve traveled far today – sitting quietly in our frames of reference.
Don’t Look At Black Holes Too Closely, They Might Disappear
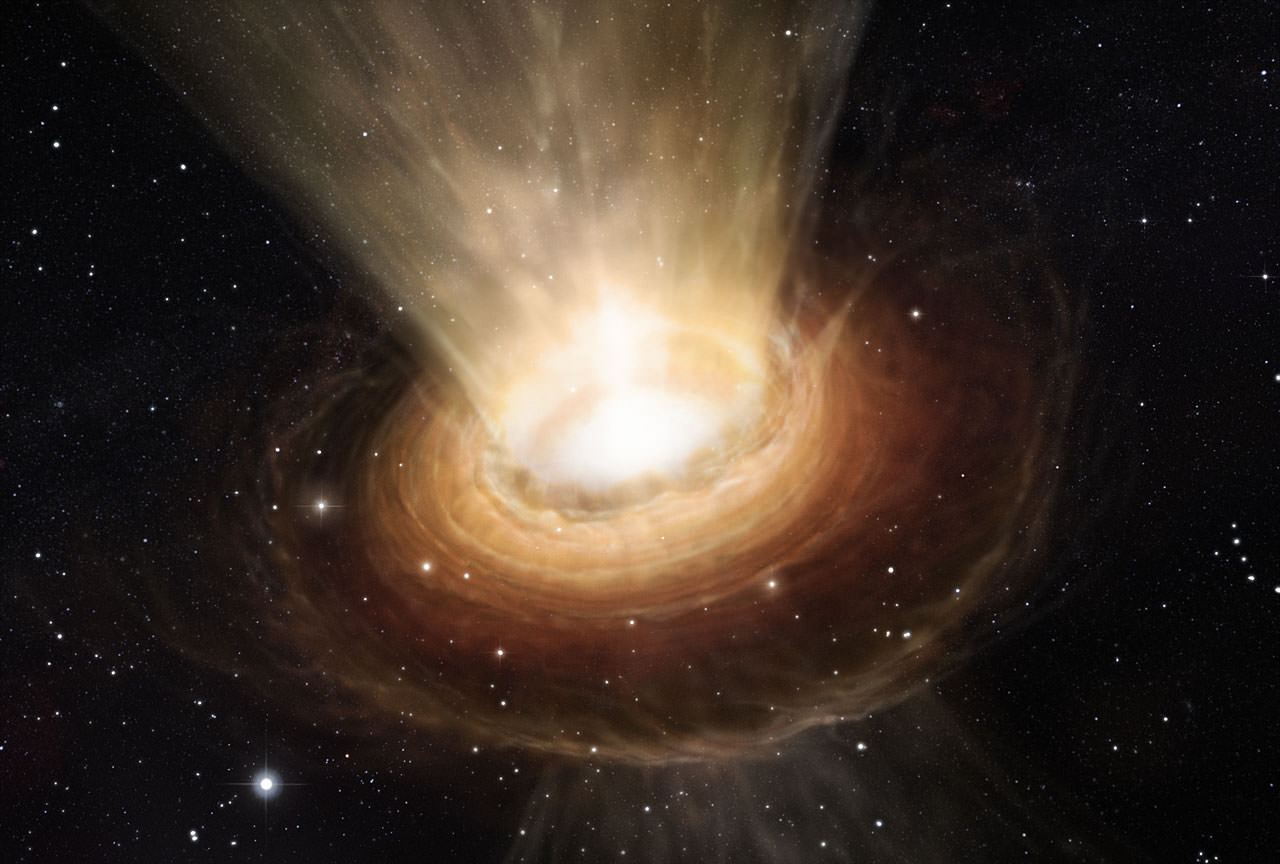
We’ve come a long way in 13.8 billion years; but despite our impressively extensive understanding of the Universe, there are still a few strings left untied. For one, there is the oft-cited disconnect between general relativity, the physics of the very large, and quantum mechanics, the physics of the very small. Then there is problematic fate of a particle’s intrinsic information after it falls into a black hole. Now, a new interpretation of fundamental physics attempts to solve both of these conundrums by making a daring claim: at certain scales, space and time simply do not exist.
Let’s start with something that is not in question. Thanks to Einstein’s theory of special relativity, we can all agree that the speed of light is constant for all observers. We can also agree that, if you’re not a photon, approaching light speed comes with some pretty funky rules – namely, anyone watching you will see your length compress and your watch slow down.
But the slowing of time also occurs near gravitationally potent objects, which are described by general relativity. So if you happen to be sight-seeing in the center of the Milky Way and you make the regrettable decision to get too close to our supermassive black hole’s event horizon (more sinisterly known as its point-of-no-return), anyone observing you will also see your watch slow down. In fact, he or she will witness your motion toward the event horizon slow dramatically over an infinite amount of time; that is, from your now-traumatized friend’s perspective, you never actually cross the event horizon. You, however, will feel no difference in the progression of time as you fall past this invisible barrier, soon to be spaghettified by the black hole’s immense gravity.
So, who is “correct”? Relativity dictates that each observer’s point of view is equally valid; but in this situation, you can’t both be right. Do you face your demise in the heart of a black hole, or don’t you? (Note: This isn’t strictly a paradox, but intuitively, it feels a little sticky.)
And there is an additional, bigger problem. A black hole’s event horizon is thought to give rise to Hawking radiation, a kind of escaping energy that will eventually lead to both the evaporation of the black hole and the destruction of all of the matter and energy that was once held inside of it. This concept has black hole physicists scratching their heads. Because according to the laws of physics, all of the intrinsic information about a particle or system (namely, the quantum wavefunction) must be conserved. It cannot just disappear.
Why all of these bizarre paradoxes? Because black holes exist in the nebulous space where a singularity meets general relativity – fertile, yet untapped ground for the elusive theory of everything.
Enter two interesting, yet controversial concepts: doubly special relativity and gravity’s rainbow.
Just as the speed of light is a universally agreed-upon constant in special relativity, so is the Planck energy in doubly special relativity (DSR). In DSR, this value (1.22 x 1019 GeV) is the maximum energy (and thus, the maximum mass) that a particle can have in our Universe.
Two important consequences of DSR’s maximum energy value are minimum units of time and space. That is, regardless of whether you are moving or stationary, in empty space or near a black hole, you will agree that classical space breaks down at distances shorter than the Planck length (1.6 x 10-35 m) and classical time breaks down at moments briefer than the Planck time (5.4 x 10-44 sec).
In other words, spacetime is discrete. It exists in indivisible (albeit vanishingly small) units. Quantum below, classical above. Add general relativity into the picture, and you get the theory of gravity’s rainbow.
Physicists Ahmed Farag Ali, Mir Faizal, and Barun Majumder believe that these theories can be used to explain away the aforementioned black hole conundrums – both your controversial spaghettification and the information paradox. How? According to DSR and gravity’s rainbow, in regions smaller than 1.6 x 10-35 m and at times shorter than 5.4 x 10-44 sec… the Universe as we know it simply does not exist.
“In gravity’s rainbow, space does not exist below a certain minimum length, and time does not exist below a certain minimum time interval,” explained Ali, who, along with Faizal and Majumder, authored a paper on this topic that was published last month. “So, all objects existing in space and occurring at a time do not exist below that length and time interval [which are associated with the Planck scale].”
Luckily for us, every particle we know of, and thus every particle we are made of, is much larger than the Planck length and endures for much longer than the Planck time. So – phew! – you and I and everything we see and know can go on existing. (Just don’t probe too deeply.)
The event horizon of a black hole, however, is a different story. After all, the event horizon isn’t made of particles. It is pure spacetime. And according to Ali and his colleagues, if you could observe it on extremely short time or distance scales, it would cease to have meaning. It wouldn’t be a point-of-no-return at all. In their view, the paradox only arises when you treat spacetime as continuous – without minimum units of length and time.
“As the information paradox depends on the existence of the event horizon, and an event horizon like all objects does not exist below a certain length and time interval, then there is no absolute information paradox in gravity’s rainbow. The absence of an effective horizon means that there is nothing absolutely stopping information from going out of the black hole,” concluded Ali.
No absolute event horizon, no information paradox.
And what of your spaghettification within the black hole? Again, it depends on the scale at which you choose to analyze your situation. In gravity’s rainbow, spacetime is discrete; therefore, the mathematics reveal that both you (the doomed in-faller) and your observer will witness your demise within a finite length of time. But in the current formulation of general relativity, where spacetime is described as continuous, the paradox arises. The in-faller, well, falls in; meanwhile, the observer never sees the in-faller pass the event horizon.
“The most important lesson from this paper is that space and time exist only beyond a certain scale,” said Ali. “There is no space and time below that scale. Hence, it is meaningless to define particles, matter, or any object, including black holes, that exist in space and time below that scale. Thus, as long as we keep ourselves confined to the scales at which both space and time exist, we get sensible physical answers. However, when we try to ask questions at length and time intervals that are below the scales at which space and time exist, we end up getting paradoxes and problems.”
To recap: if spacetime continues on arbitrarily small scales, the paradoxes remain. If, however, gravity’s rainbow is correct and the Planck length and the Planck time are the smallest unit of space and time that fundamentally exist, we’re in the clear… at least, mathematically speaking. Unfortunately, the Planck scales are far too tiny for our measly modern particle colliders to probe. So, at least for now, this work provides yet another purely theoretical result.
The paper was published in the January 23 issue of Europhysics Letters. A pre-print of the paper is available here.
Does Free Will Exist? Ancient Quasars May Hold the Clue.

Do you believe in free will? Are people able to decide their own destinies, whether it’s on what continent they’ll live, who or if they’ll marry, or just where they’ll get lunch today? Or are we just the unwitting pawns of some greater cosmic mechanism at work, ticking away the seconds and steering everyone and everything toward an inevitable, predetermined fate?
Philosophical debates aside, MIT researchers are actually looking to move past this age-old argument in their experiments once and for all, using some of the most distant and brilliant objects in the Universe.
Rather than ponder the ancient musings of Plato and Aristotle, researchers at MIT were trying to determine how to get past a more recent conundrum in physics: Bell’s Theorem. Proposed by Irish physicist John Bell in 1964, the principle attempts to come to terms with the behavior of “entangled” quantum particles separated by great distances but somehow affected simultaneously and instantaneously by the measurement of one or the other — previously referred to by Einstein as “spooky action at a distance.”
The problem with such spookiness in the quantum universe is that it seems to violate some very basic tenets of what we know about the macroscopic universe, such as information traveling faster than light. (A big no-no in physics.)
(Note: actual information is not transferred via quantum entanglement, but rather it’s the transfer of state between particles that can occur at thousands of times the speed of light.)
Read more: Spooky Experiment on ISS Could Pioneer New Quantum Communications Network
Then again, testing against Bell’s Theorem has resulted in its own weirdness (even as quantum research goes.) While some of the intrinsic “loopholes” in Bell’s Theorem have been sealed up, one odd suggestion remains on the table: what if a quantum-induced absence of free will (i.e., hidden variables) is conspiring to affect how researchers calibrate their detectors and collect data, somehow steering them toward a conclusion biased against classical physics?
“It sounds creepy, but people realized that’s a logical possibility that hasn’t been closed yet,” said David Kaiser, Germeshausen Professor of the History of Science and senior lecturer in the Department of Physics at MIT in Cambridge, Mass. “Before we make the leap to say the equations of quantum theory tell us the world is inescapably crazy and bizarre, have we closed every conceivable logical loophole, even if they may not seem plausible in the world we know today?”
So in order to clear the air of any possible predestination by entangled interlopers, Kaiser and MIT postdoc Andrew Friedman, along with Jason Gallicchio of the University of Chicago, propose to look into the distant, early Universe for sufficiently unprejudiced parties: ancient quasars that have never, ever been in contact.
According to a news release from MIT:
…an experiment would go something like this: A laboratory setup would consist of a particle generator, such as a radioactive atom that spits out pairs of entangled particles. One detector measures a property of particle A, while another detector does the same for particle B. A split second after the particles are generated, but just before the detectors are set, scientists would use telescopic observations of distant quasars to determine which properties each detector will measure of a respective particle. In other words, quasar A determines the settings to detect particle A, and quasar B sets the detector for particle B.
By using the light from objects that came into existence just shortly after the Big Bang to calibrate their detectors, the team hopes to remove any possibility of entanglement… and determine what’s really in charge of the Universe.
“I think it’s fair to say this is the final frontier, logically speaking, that stands between this enormously impressive accumulated experimental evidence and the interpretation of that evidence saying the world is governed by quantum mechanics,” said Kaiser.
Then again, perhaps that’s exactly what they’re supposed to do…
The paper was published this week in the journal Physical Review Letters.
Source: MIT Media Relations
Want to read more about the admittedly complex subject of entanglement and hidden variables (which may or may not really have anything to do with where you eat lunch?) Click here.
Don’t Tell Bones: Are We One Step Closer to “Beaming Up?”
It’s a crazy way to travel, spreading a man’s molecules all over the Universe…
While we’re still a very long way off from instantly transporting from ship to planet à la Star Trek, scientists are still relentlessly working on the type of quantum technologies that could one day make this sci-fi staple a possibility. Just recently, researchers at the University of Cambridge in the UK have reported ways to simplify the instantaneous transmission of quantum information using less “entanglement,” thereby making the process more efficient — as well as less error-prone.
(Because nobody wants a transporter mishap.)
In a paper titled Generalized teleportation and entanglement recycling, Cambridge researchers Sergii Strelchuk, Michal Horodecki and Jonathan Oppenheim investigate a couple of previously-developed protocols for quantum teleportation.
“Teleportation lies at the very heart of quantum information theory, being the pivotal primitive in a variety of tasks. Teleportation protocols are a way of sending an unknown quantum state from one party to another using a resource in the form of an entangled state shared between two parties, Alice and Bob, in advance. First, Alice performs a measurement on the state she wants to teleport and her part of the resource state, then she communicates the classical information to Bob. He applies the unitary operation conditioned on that information to obtain the teleported state.” (Strelchuk et al.)
In order for the teleportation to work, the process relies on entanglement — the remote connection between particles or individual bits of information regardless of the physical space separating them. This was what Einstein referred to as “spooky action at a distance.” But getting particles or information packets entangled is no simple task.
“Teleportation crucially depends on entanglement, which can be thought as a ‘fuel’ powering it,” Strelchuk said in an article on ABC Science. “This fuel… is hard to generate, store and replenish. Finding a way to use it sparingly, or, ideally, recycling it, makes teleportation potentially more usable.”
Read: Beam Me Up, Obama: Conspiracy Theory Claims President Teleported to Mars
Considering the sheer amount of information that makes up the also-difficult-to-determine state of a single object (in the case of a human, even simplistically speaking, about 10^28 kilobytes worth of data) you’re obviously going to want to keep the amount of entanglement fuel needed at a minimum.
Of course, we’re not saying we can teleport red-shirted security officers anywhere yet. But if.
Still, with a more efficient method to reduce — and even recycle — entanglement, Strelchuk and his team are bringing us a little closer to making quantum computing a reality. And it may very well take the power of a quantum computer to even make the physical teleportation of large-scale objects possible… once the technology becomes available.
“We are very excited to show that recycling works in theory, and hope that it will find future applications in areas such as quantum computation,” said Strelchuk. “Building a quantum computer is one of the great challenges of modern physics, and it is hoped that the new teleportation protocol will lead to advances in this area.”
(I’m sure Dr. McCoy would still remain skeptical.)
You can find the team’s full paper here (chock full of maths!) and read the article on ABC Science by Stephen Pincock here.
Transporter room image from TOS “Obsession” episode. © 2013 CBS Studios Inc. All Rights Reserved.