The Universe is an extremely big place. As astronomers looked farther into space over the centuries, and deeper into the past, they came to understand just how small and insignificant our planet and our species seem by comparison. At the same time, ongoing investigations into electromagnetism and distant stars led scientists to deduce what the the speed of light is – and that it is the fastest speed obtainable.
As such, astronomers have taken to using the the distance light travels within a single year (aka. a light year) to measure distances on the interstellar and intergalactic scale. But how far does light travel in a year? Basically, it moves at a speed of 299,792,458 meters per second (1080 million km/hour; 671 million mph), which works out to about 9,460.5 trillion km (5,878.5 trillion miles) per year.
The Speed of Light:
Calculating the speed of light has been a preoccupation for scientists for many centuries. And prior to the 17th century, there was disagreement over whether the speed of light was finite, or if it moved from one spot to the next instantaneously. In 1676, Danish astronomer Ole Romer settled the argument when his observations of the apparent motion of Jupiter’s moon Io revealed that the speed of light was finite.
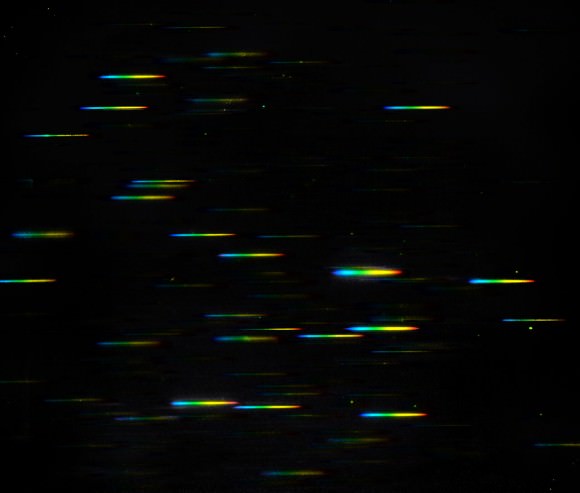
From his observations, famed Dutch astronomer Christiaan Huygens calculated the speed of light at 220,000 km/s (136,701 mi/s). Over the course of the nest two centuries, the speed of light was refined further and further, producing estimates that ranged from about 299,000 to 315,000 km/s (185,790 to 195,732 mi/s).
This was followed by James Clerk Maxwell, who proposed in 1865 that light was an electromagnetic wave. In his theory of electromagnetism, the speed of light was represented as c. And then in 1905, Albert Einstein proposed his theory of Special Relativity, which postulated that the speed of light (c) was constant, regardless of the inertial reference frame of the observer or the motion of the light source.
By 1975, after centuries of refined measurements, the speed of light in a vacuum was calculated at 299,792,458 meters per second. Ongoing research also revealed that light travels at different wavelengths and is made up of subatomic particles known as photons, which have no mass and behave as both particles and waves.
Light-Year:
As already noted, the speed of light (expressed in meters per second) means that light travels a distance of 9,460,528,000,000 km (or 5,878,499,817,000 miles) in a single year. This distance is known as a “light year”, and is used to measure objects in the Universe that are at a considerable distances from us.

For example, the nearest star to Earth (Proxima Centauri) is roughly 4.22 light-years distant. The center of the Milky Way Galaxy is 26,000 light-years away, while the nearest large galaxy (Andromeda) is 2.5 million light-years away. To date, the candidate for the farthest galaxy from Earth is MACS0647-JD, which is located approximately 13.3 billion light years away.
And the Cosmic Microwave Background, the relic radiation which is believed to be leftover from the Big Bang, is located some 13.8 billion light years away. The discovery of this radiation not only bolstered the Big Bang Theory, but also gave astronomers an accurate assessment of the age of the Universe. This brings up another important point about measuring cosmic distances in light years, which is the fact that space and time are intertwined.
You see, when we see the light coming from a distant object, we’re actually looking back in time. When we see the light from a star located 400 light-years away, we’re actually seeing light that was emitted from the star 400 years ago. Hence, we’re seeing the star as it looked 400 years ago, not as it appears today. As a result, looking at objects billions of light-years from Earth is to see billions of light-years back in time.
Yes, light travels at an extremely fast speed. But given the sheer size and scale of the Universe, it can still take billions of years from certain points in the Universe to reach us here on Earth. Hence why knowing how long it takes for light to travel a single year is so useful to scientists. Not only does it allow us to comprehend the scale of the Universe, it also allows us to chart the process of cosmic evolution.
We have written many articles about the speed of light here at Universe Today. Here’s How Far is a Light Year?, What is the Speed of Light?, How Much Stuff is in a Light Year?, How Does Light Travel?, and How Far Can You See in the Universe?
Want more info on light-years? Here’s an article about light-years for HowStuffWorks, and here’s an answer from PhysLink.
We’ve also recorded an episode of Astronomy Cast on this topic. Listen here, Episode 10: Measuring Distance in the Universe.
Sources: