[/caption]
The Milky Way is pretty hard to map, given that we live inside of it and have to peer through all of the dust and gas that lie inside the disk. Though we can’t get a picture of our galaxy from outside, we can create images and maps from computer modeling of the stars we see in the disk.
The Milky Way – until recently – was thought to be a barred spiral with four star-forming arms, named Norma, Scutum-Centaurus, Sagittarius and Perseus. In June of 2008, images from NASA’s Spitzer Space Telescope revealed that the Milky Way is a barred spiral with only two major arms, demoting the Sagittarius and Norma arms to minor arms. The Sun lies in a minor arm, named the Orion Arm, or Orion Spur, sandwiched between the Sagittarius minor arm and Perseus arm.
Our galaxy is a large disk approximately 100,000 light years across. There’s a bulge in the center that is 12,000-16,000 light years thick, and is home to a black hole named Sagittarius A*. Other areas of the disk range between 2,300 and 2,600 light years in thickness.
The image above is a representation of what the Milky Way would look like from above. Of course, there are plenty of maps of the Milky Way as we see it from the Earth. Also, you can go outside on a clear night and see it splashed across the sky. Below is an image in the infrared, with the various regions marked (named because of the constellation in which they lie).
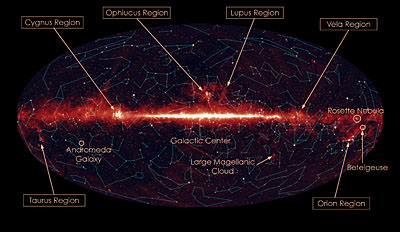
Plenty of maps are available all over the web in a variety of spectrum. Here’s a gallery of 9 images of the Milky Way in different spectra to get you started, and An Atlas of the Universe has maps of the Milky Way and Universe from different perspectives and distances. If you’re looking for interactive maps of the sky and Milky Way, Sky-Map.org, Google Sky and the downloadable Stellarium are all great resources to familiarize yourself with our Galaxy.
If you’re interested in learning more about other aspects of the Milky Way, Astronomy Cast has a whole episode devoted to it. You can also check out the rest of our resources in the Milky Way section of the Guide to Space.