This Saturday September 22, 2012 marks the 3rd annual International Observe the Moon Night (InOMN), when people all over the world will gather to observe the Moon. But what do you do the rest of the year? Luckily, in today’s internet age, there is a great deal of lunar data, from a range of missions, available on-line for you to look at. Also, some great tools have been developed that make data easy to access, put into context, and interpret, giving everyone the power to explore the Moon like a scientist. All you need to do it click on the URL and you’re off…
InOMN was originally started in as a celebration of the wonderful lunar data that was being returned by missions such as the Lunar Reconaissance Orbiter, Chandrayaan-1, and other spacecraft. Since then it has grown to phenomenal proportions, with hundreds of individual events hosted literally all over the world. To learn more about InOMN, or to find the event nearest you, visit the InOMN website.
But what do you do if there is no event being hosted near you, or if the weather turns cloudy in your geographic region? You can always join the CosmoQuest InOMN Hangout on Google+.
For more information about InOMN, listen to a 365 Days of Astronomy podcast on this year’s event.
However, a true passion and interest in the Moon is not a one day thing. What if you want to look at the Moon on some other day, or see details that are too small to be resolved by even the largest telescopes on Earth? As it happens, data from those same missions that inspired the very first InOMN is very easy for the average person to see, any time they want to. Lunar Reconnaissance Orbiter Camera (LROC) data from the Lunar Reconnaissance Orbiter and Moon Mineralogy Mapper (M3) data from the Chandrayaan-1 spacecraft can be accessed on-line using the ACT-REACT Quick Map tool.
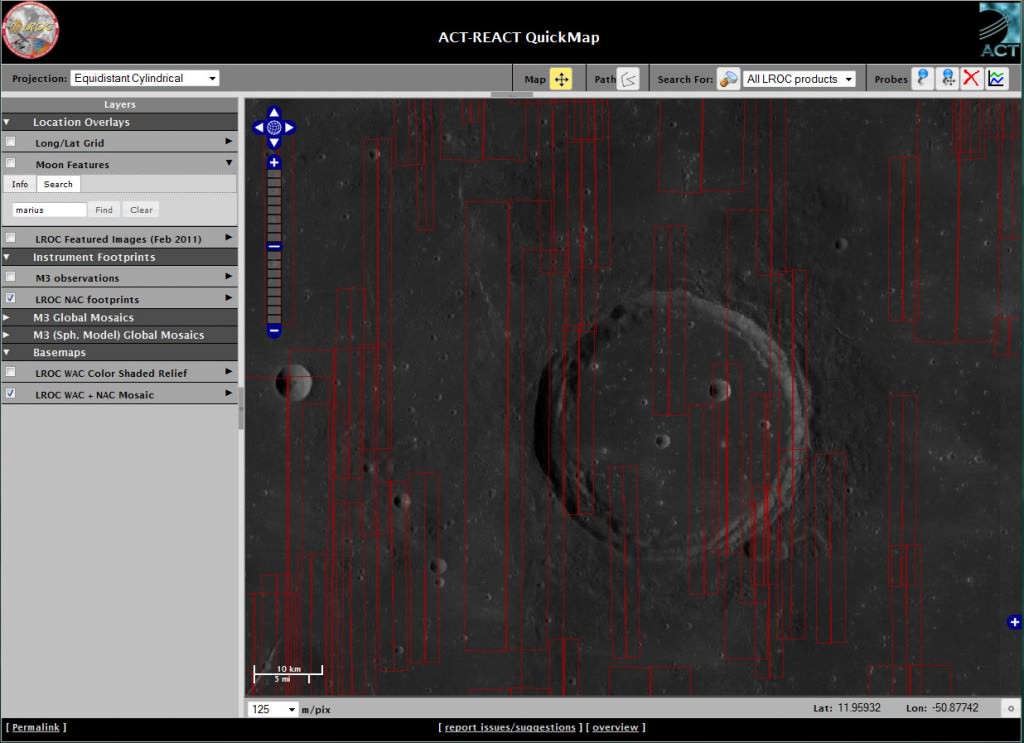
This LROC version of the ACT-REACT Quick Map tool (there is also a MESSENGER version for Mercury data) was originally developed by the LROC team to place skinny little strips of LROC Narrow Angle Camera data into context on the Moon, and to help with targeting for further high resolution data collection. They partnered with software firm Applied Coherent Technology (ACT) to create this relatively user friendly on-line tool, and then made it accessible for anyone who wants to use it!
The interface of the ACT-REACT Quick Map tool is fairly intuitive. If you have used Google Maps, you should be able to navigate your way around fairly quickly. For more details on the available features, check out the LROC data user tutorial and the M3 data user tutorial. Though, one of the first things you might want to know how to do is to turn off the bright colours that represent elevation (uncheck the LROC WAC Color Shaded Relief checkbox). This shaded relief layer is great when you want to understand the topography of fairly large features, but is more distracting than helpful when looking at highest resolution data.
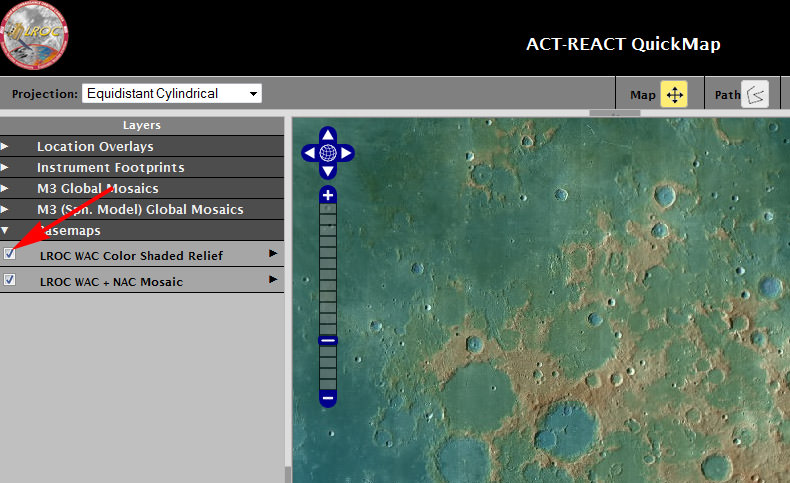
The most exciting thing about the ACT-REACT Quick Map tool is that it makes these amazing lunar data sets available to the public in a way that was never possible before. Anyone sitting at their computer at home can study the Moon, viewing large lunar features, like impact basins and maria, and then zooming into to see details as small as their desk. This kind of technological advance opens the door for every enthusiast to conduct their own personal explorations of the Moon, and gives them an opportunity to see and think like the scientists who are currently working with this data to discover new and exciting information about our Moon.
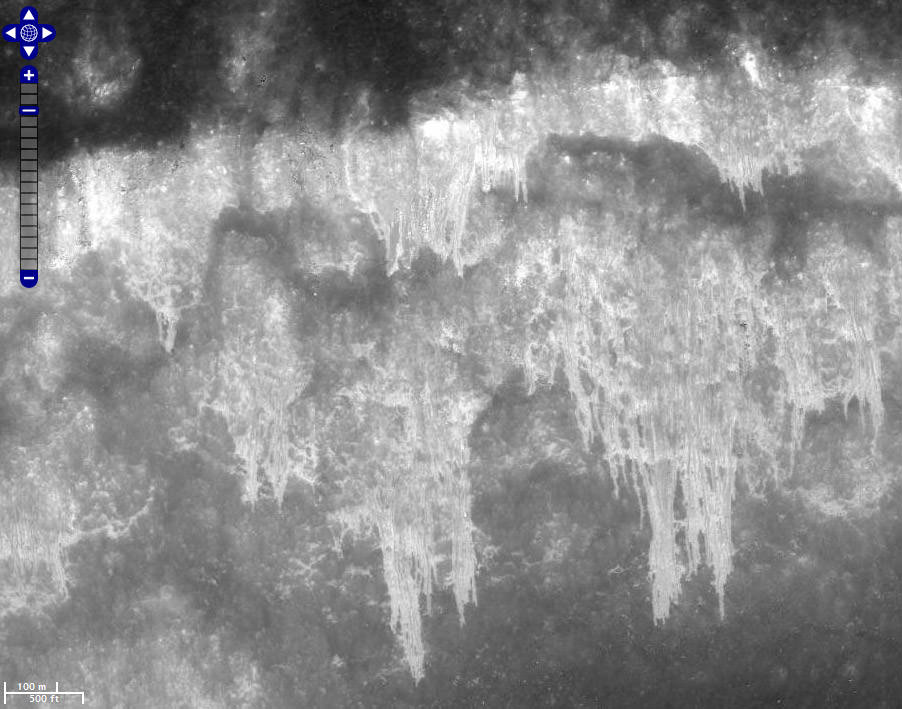
So, after International Observe the Moon Night is over, don’t wait until next year to look at the Moon again. Head over to ACT-REACT Quick Map and start exploring!