Antoine Henri Becquerel discovered radioactivity completely by accident when he exposed a chunk of uranium to a photographic plate. This opened up a whole new field of research to uncover the source of the mysterious energy.
Continue reading “Astronomy Cast Ep. 373: Becquerel Experiment (Radiation)”
What is Beta Radiation?
Beta radiation is radiation due to beta particles, which are electrons (or, sometimes, positrons); mostly, when you come across the words ‘beta radiation’, what is meant is what is produced by beta decay (radioactive decay which produces beta particles … either electrons or positrons).
Within a few years of Becquerel’s discovery of radioactivity (in 1896), its heterogeneous nature was discovered … and the three (then) known components given the memorable names alpha radiation, beta radiation, and gamma radiation. And, in 1900, Becquerel showed that beta radiation was composed of particles which have the same charge-to-mass ratio as electrons (which had been discovered only a few years’ earlier). The realization – by Irène and Frédéric Joliot-Curie, in 1934 – that some beta radiation is composed of positrons, rather than electrons, had to wait until positrons themselves were discovered (in 1932).
Some fun facts about beta radiation:
* beta radiation is in between alpha and gamma in terms of its penetrating power; typically it goes a meter or so in air
* like all kinds of radioactive decay, beta decay occurs because the final state of the nucleus (the one decaying) has a lower energy than the initial one (the difference is the energy of the emitted beta particle and neutrino)
* beta decay involves only the weak interaction (or force), unlike alpha and gamma decay
* the key to the specifics of beta decay is the emission of a neutrino (or antineutrino), postulated by Pauli (in 1931) and combined into a model by Fermi, in 1934 (though it wasn’t until 1956 that the neutrino was detected, and the 1960s for the existence of carriers of the weak force – the three bosons W–, W+, and Z0 – to be hypothesized).
* beta radiation has the characteristics we observe it to have because key constants in the weak interaction have the values they have (no theory in physics predicts what those values are … yet); had those values been just a teensy bit different in the early universe, we would not be here today (this is part of an idea called the anthropic principle).
Here are some of the Universe Today stories that are related to beta radiation New Insights on Magnetars, Superstrings Could Be Detectable As They Decay, and Don’t ‘Supermassive’ Me: Black Holes Regulate Their Own Mass.
Two Astronomy Cast episodes are well worth a listen, as they provide further insights into beta radiation The Strong and Weak Nuclear Forces, and Nucleosynthesis: Elements from Stars.
Beta Particles
Beta particles are electrons (symbol β–), or positrons (symbol β+), emitted in beta decay (a kind of radioactivity); beta radiation in other words. Sometimes ‘beta particles’ refers to high energy electrons, irrespective of their source (e.g. the beta particles in the Van Allen radiation belts around the Earth; very few are produced by beta decay).
Of the three kinds of radioactivity (alpha, beta – both of which are particles – and gamma (which is electromagnetic radiation)), beta particles have intermediate penetrating power.
Beta particles have an important role in medicine … as diagnostic tools, to treat some diseases (notably various cancers, particularly via radionuclide therapy), in biochemical analysis, etc. For example, 18F (the fluorine-18 isotope) is used as a positron (β+) emitter in positron emission tomography (PET).
Beta particles – or rather the weak interaction which is the cause of their emission – were crucial in Big Bang Nucleosynthesis … as the early universe cooled, reactions between the protons, neutrons, electrons, and photons produced many light nuclides, but the balance between many reactions left only hydrogen, deuterium, helium-3, helium-4, and lithium-7 when the universe became too cool for any nuclear reactions to continue (of course, isolated neutrons and unstable nuclides – such as tritium – were also left, but they decayed well before the time of cosmic microwave background).
Fast forward to today … beta (β+) particles from the decay of potassium-40 is one source of internal heat for the Earth – giving us plate tectonics, its magnetic field, etc – … the decay of carbon-14 and beryllium-10 (both of which produce beta (β–) particles) provide us with tools to do radioactive (or radiometric) dating (these are two of the nuclides produced by cosmic ray spallation).
Beta Particle Radiation is a good, introductory webpage (from the University of California, Davis), and Weak Interactions explains how beta particles and the weak (nuclear) force are related (from SLAC’s Virtual Visitor Center)
Universe Today has several stories which cover the role of beta particles in astronomy; for example A Prototype Detector for Dark Matter in the Milky Way, and Fermilab Putting the Squeeze on Higgs Boson.
The Strong and Weak Nuclear Forces, and Nucleosynthesis: Elements from Stars are two Astronomy Cast episodes which will help you understand beta particles better.
Beta Decay
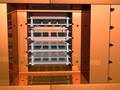
[/caption]
Beta decay is when an unstable atomic nucleus decays (radioactively) by emitting a beta particle; when the beta particle is an electron, it is β– decay, and when a positron, β+ decay.
Beta rays, as a distinct component of the rays given off in radioactivity, were discovered by Rutherford, in 1899, just a few years after radioactivity itself was discovered (in 1896). However, this is beta minus decay … the discovery of beta plus decay (by Irène and Frédéric Joliot-Curie, in 1934) came after the discovery of the positron (in cosmic rays, in 1932) and the (then) controversial ‘invention’ of the neutrino (by Pauli, in 1931) to account for the continuous energy spectrum of electrons in beta decay. It was also in 1934 that Fermi published – in Italian and German (Nature considered the idea too speculative!!) – his theory of beta decay (for more details on this, check out this Hyperphysics page).
In beta minus decay, a neutron changes into a proton, antineutrino, and electron; this conversion is due to the weak interaction (or weak force) … a down quark (in the neutron) becomes an up quark and emits a W– boson (one of three bosons which mediate the weak interaction), which then decays into an electron and an antineutrino.
Beta plus decay – which is also known as inverse beta decay – involves the conversion of a proton to a neutron, positron, and neutrino.
So why do isolated neutrons decay (but those in stable nuclei, and those in neutron stars, don’t)? And why are isolated protons stable, but those in certain radioactive nuclei not? It’s all down to energy … if one state (an isolated neutron, say) has a higher energy than another (proton plus electron plus antineutrino), then the first will decay into the second (the baryon number of the two states must be the same, ditto lepton number, and so on).
There is also a rare double beta decay, in which two beta particles are emitted; it has been observed, in some unstable isotopes, as predicted. There is one kind of double beta decay – called neutrino-less double beta decay (the image above is from the COBRA Project, one study of this) – which is being studied intensely (though no such decay has yet been observed), because it may be one of the very few easily opened windows into physics beyond the Standard Model (see this WIPP page for more details).
Berkeley Lab has a neat Guide to the Nuclear Wallchart (subtitled “You don’t need to be a Nuclear Physicist to understand Nuclear Science“!) on beta decay, and this Ohio University page – Alpha and beta decay – puts more technical meat on the bare overview bones.
Pushing the Polite Boundaries of Science About Dark Matter is a Universe Today story which has a tangential reference to beta decay (it’s in the comments!).
Are there relevant Astronomy Cast episodes? Sure! Nucleosynthesis: Elements from Stars, The Strong and Weak Nuclear Forces, and Antimatter.
Source:
Wikipedia
What is Alpha Radiation?
Alpha radiation is another name for the alpha particles emitted in the type of radioactive decay called alpha decay. Alpha particles are helium-4 (4He) nuclei.
Radioactivity was discovered by Becquerel, in 1896 (and one of the units of radioactivity – the becquerel – is named after him); within a few years it was discovered (Rutherford gets most of the credit, though others contributed) that there are actually three kinds of radioactivity, which were given the exciting names alpha (radiation), beta (radiation), and gamma (radiation; there are some other, rare, kinds of radioactive decay, the most important being positron, or positive beta). Rutherford (with some help) worked out that alpha radiation is actually the nuclei of helium … by allowing alpha radiation to go through the thin walls of an evacuated glass tube, and later analyzing the gas in the tube spectroscopically).
Some fun facts about alpha radiation:
* alpha radiation is the least penetrating (of alpha, beta, and gamma); typically it goes no more than a few cm in air
* like all kinds of radioactive decay, alpha decay occurs because the final state of the nucleus (the one decaying) has a lower energy than the initial one (the difference is the energy of the emitted alpha particle, both its binding energy and its kinetic energy)
* alpha decay involves both strong and electromagnetic interactions (or forces), unlike beta and gamma decay
* the key to the specifics of alpha decay is the quantum effect called tunneling; Gamow worked this out, in 1928
* only heavier nuclides can undergo alpha decay; the lightest are light isotopes of tellurium
* alpha radiation played a star role in the development of our understanding of the nature of atoms … Rutherford, in 1909, aimed a beam of alpha radiation at a piece of thin gold foil, and counted the number of particles which were deflected at each angle; from this he deduced that the atom has a very small nucleus (with all the positive charge, and nearly all its mass).
For more background on alpha radiation, check out the Jefferson Lab’s What are alpha rays? How are they produced?.
There are many ways alpha radiation can turn up in Universe Today articles; for example, in NASA May Have to Revamp Science Plans Without RTGs, alpha radiation is essential to RTGs; and in Opportunity Rover Sidelined by Charged Particle Hit, alpha radiation is what’s used to help determine the elemental composition of samples.
Nucleosynthsis: Elements from Stars and Cosmic Rays are two Astronomy Cast episodes which also cover alpha radiation.
Source: Wikipedia
Radioactive Hot Spots on Earth’s Beaches May Have Sparked Life
We’ve heard about life being created in a puddle of primordial chemical soup, sparked by lightning strikes, or organic molecules falling to Earth from comets or planets, such as Mars. But now, there is an alternative. Early Earth was radioactive; the Moon also had a lower orbit, generating strong tidal forces. Due to the close proximity to abundant water, radioactive beaches may have possessed all the essential ingredients for organic compounds, and eventually life, to thrive.
Research by the University of Washington, Seattle, suggests that perhaps the highly radioactive environment of Earth some 4 billion years ago may have been the ideal time for life to form. The orbit of the Moon also had a part to play in this offbeat theory.
Through strong tidal forces by a Moon that orbited far closer to the Earth than it does today, radioactive elements accumulated on the beaches could be gravitationally sorted. The chemical energy in these beach hot spots was probably high enough to allow self-sustaining fission processes (which occurs in natural concentrations of uranium). The main product from fission is heat, therefore powering chemical processes and the generation of organic, life-giving compounds.
“Amino acids, sugars and [soluble] phosphate can all be produced simultaneously in a radioactive beach environment.” – Zachary Adam, an astrobiologist at the University of Washington Seattle.
This is a hard theory to understand, it is well known that radioactivity breaks down organic molecules and causes a whole host of problems for us carbon-based creatures. But in the early Earth, devoid of plants and animals, radioactive processes may have provided energy for life to begin in the first place.
This theory also partially explains why life may be a very rare occurrence in the universe: there must be the correct concentration of radioactive elements, on the surface of a water-dominated developing planet, with tidal forces supplied by a closely orbiting stellar body. The Earth may, after all, be unique.
Source: Telegraph.co.uk