E = mc². It’s one of the most basic and fundamental equations throughout astrophysics. But it does more than suggest that mass and energy are interconnected, it implies that light can be physically transformed into matter.
But can it really — physically — be done? Scientists proposed the theory more than 80 years ago, but only today have they paved the way to make this transformation routinely on Earth.
The concept calls for a new kind of photon-photon collider. It sounds like science fiction, but it could be turned into reality with existing technology.
“Although the theory is conceptually simple, it has been very difficult to verify experimentally,” said lead researcher Oliver Pike from London’s Imperial College in a press release. “We were able to develop the idea for the collider very quickly, but the experimental design we propose can be carried out with relative ease.”
In 1934, two physicists Gregory Breit and John Wheeler proposed that it should be possible to turn light into matter by smashing together only two photons, the fundamental particles of light, to create an electron and a positron. It was the simplest method of turning light into matter ever predicted, but it has never been observed in the laboratory.
Past experiments have required the addition of massive high-energy particles. We’ve seen from the development of nuclear weapons and fission reactors that a tiny amount of matter can yield a tremendous amount of energy. So it seems Breit and Wheeler’s theory would require the opposite effect: tremendous amounts of energy from photons to yield a tiny amount of matter.
This experiment will be a first in that it doesn’t require the addition of massive high-energy particles. It will be performed purely from photons.
The concept calls for using a high-intensity laser to speed up electrons to just below the speed of light, and then smash them into a slab of gold to create a beam of photons a billion times more energetic than visible light. At the same time, another laser beam would be blasted onto a hohlraum — a small gold container meaning “empty can” in German — that would create a radiation field with photons buzzing inside.
The initial photon beam would be directed into the center of the hohlraum. When the photons from the two sources collide, some would be converted into pairs of electrons and positrons. A detector would then pick up the signatures form the matter and antimatter as they flew out of the container.
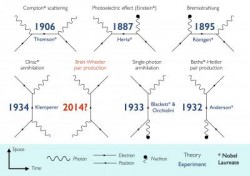
“Within a few hours of looking for applications of hohlraums outside their traditional role in fusion energy research, we were astonished to find they provided the perfect conditions for creating a photon collider,” Pike said. “The race to carry out and complete the experiment is on!”
The demonstration, if carried out successfully, would be a new type of high-energy physics experiment. It would complete physicists’ list of the fundamental ways in which light and matter interact, and both recreate a process that was important 100 seconds after the Big Bang and a process visible in gamma ray bursts, the most powerful explosions in the cosmos.
The paper has been published in Nature Photonics.