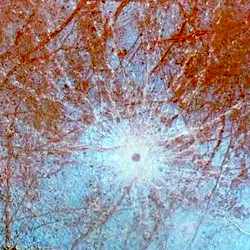
Europa. Image credit: NASA Click to enlarge
The discovery that Jupiter’s moon Europa most likely has a cold, salty ocean beneath its frozen icy crust has put Europa on the short list of objects in our solar system that astrobiologists would like to study further. At the Earth System Processes II conference in Calgary, Canada, Ron Greeley, planetary geologist and professor of geology at Arizona State University in Phoenix, Arizona, gave a talk summing up what is known about Jupiter and its moons, and what remains to be discovered.
There have been six spacecraft that have explored the Jupiter system. The first two were Pioneer spacecraft in the 1970s that flew by the Jupiter system and made some brief observations. Those were followed by the Voyager I and II spacecraft, which gave us our first detailed views of the Galilean satellites. But most of the information we have has come from the Galileo mission. More recently, there was a flyby of the Cassini spacecraft, that went by Jupiter and made observations on its way to Saturn, where it is currently in operation. But nearly everything we know about the geology of the Jupiter system, and in particular the Galilean satellites (Io, Europa, Ganymede and Callisto), came from the Galileo mission. Galileo gave us an incredible wealth of information that we’re still in the process of analyzing today.
There are four Galilean satellites. Io, the innermost, is volcanically the most active object in the solar system. It derives its internal energy from tidal stressing in the interior, as it is being pushed-pulled between Europa and Jupiter. The explosive volcanism we see there is very impressive. There are plumes that are ejected some 200 kilometers (124 miles) above the surface. We also see effusive volcanism in the form of lava flows erupting onto the surface. These are very high-temperature, very fluid flows. On Io we see these flows extending for hundreds of kilometers across the surface.
All of the Galilean satellites are in elliptical orbits, which means that sometimes they’re closer to Jupiter, other times they’re farther away, and they’re being pushed-pulled by their neighbors. That generates internal friction to sufficient levels, in the case of Io, to melt the interior and “drive” the volcanoes. The same processes are taking place on Europa. And there is a possibility of silicate volcanism taking place beneath the icy crust on Europa.
Ganymede is the largest satellite in the solar system. It has an outer icy shell. We think that it has a sub-ice ocean of liquid water over a silicate core and perhaps a small internal metallic core. Ganymede has been subjected to geologic processes since its formation. It has a complex history, dominated by tectonic processes. We see a combination of very old features and very young features. We can see complex facture patterns on its surface that crosscut older fracture patterns. The surface is fractured into blocks that have been shifted about on the overriding, apparently liquid, interior. We also see the impact history dating from the period of early bombardment. Unscrambling the tectonic history of Ganymede is a work in process.
Callisto is the outermost of the Galilean satellites. It, too, has been subjected to impact bombardment, reflecting the early accretion history of the solar system in general, and the Jupiter system in particular. The surface is dominated by craters of all sizes. But we were surprised by the apparent lack of very tiny impact craters. We see very tiny impact craters on its neighbor, Ganymede; we don’t see them on Callisto. There is some process, we think, that is erasing the small craters – but only in selected areas on the moon. This is a mystery that has not been resolved: What is the process that is removing the tiny craters in some areas, or alternatively, might they not have formed there for some reason to begin with? Again, this is a topic of ongoing research.
What I want to talk about primarily, though, is Europa. Europa is about the size of Earth’s moon. It is primarily a silicate object, but it has an outer shell of H2O, the surface of which is frozen. The total volume of water that covers its silicate interior exceeds all of the water on Earth. The surface of that water is frozen. The question is: What’s beneath that frozen shell? Is there solid ice all the way to the bottom, or is there a liquid ocean? We think there is liquid water beneath the icy crust, but we don’t really know that for sure. Our ideas are based on models, and like all models, they are subject to further study.
The reason we think that there is a liquid ocean on Europa is from the behavior of the induced magnetic field around Europa that was measured by the magnetometer on Galileo. Jupiter has an enormous magnetic field. It, in turn, induces a magnetic field, not only on Europa, but also on Ganymede and Callisto. The way that induced magnetic field behaves is consistent with the presence of a subsurface salty liquid ocean, not just on Europa, but also on Ganymede and Callisto.
We do know that the surface is water ice. We know that there are non-ice components present, which includes various salts. And we know that the surface has been geologically processed: it has been fractured, healed, broken up repeatedly. We also see relatively few impact craters on the surface. That indicates that the surface is geologically young. Europa could even be geologically active today. Images of one region, in particular, show a surface that has been severely broken up. The icy plates have been broken apart and shifted into new positions. Material has oozed between the cracks, then apparently frozen, and we think that this could be one of the places where there was upwelling material, perhaps driven by the tidal heating I talked about earlier.
We tend to forget the scale of things in the planetary sciences. But these icy blocks are huge. When we think about future exploration, we would like to get down on the surface and make certain key measurements. So we have to think about spacecraft systems that could land in this kind of terrain. Because it is these places that might have material derived from below the ice, they are the highest priority for exploration. And yet, as is often the case in planetary exploration, the most interesting places are the most difficult to get to.
So what would we like to know? First and most fundamental is the “ocean notion.” Does liquid water exist or not? Is the ice shell thick or thin? If there is an ocean there, how thick is that icy crust? This is very important to know when we think about exploring a possible liquid ocean on Europa: If we want to get into the ocean, how deep must we go through the ice? What is the age of the surface? We say “young,” but that’s only a relative term. Is it thousands, hundreds of thousands, millions, or even billions of years old? The models allow for quite a spread in ages, based on the impact crater frequency. What are the environments there today that are favorable for astrobiology? And what were the environments in the past? Were they the same, or have they changed through time? The answers to these questions require new data.
Another thing that drives our interest in exploring the Galilean satellites is trying to understand their geological histories. To some extent, the diversity that we see, from Io to Europa to Ganymede and Callisto, can be linked to the amount of tidal energy that’s driving the system. Maximum tidal energy drives the volcanism that is so dominant on Io. At the other extreme, very little tidal energy on Callisto results in the preservation of the impact-cratering record. Europa and Ganymede are in between these two extreme cases.
The total surface area of the three icy moons of Jupiter (Europa, Ganymede and Callisto) is greater than the surface area of Mars, and, in fact, is about equivalent to the entire land surface of Earth. So when we discuss the exploration of the icy Galilean satellites, there is a lot of terrain to cover.
As for future exploration, let me share a little history. Three years ago, NASA established the Prometheus project. The Prometheus project involves the development of nuclear power and nuclear propulsion, something that had not been considered seriously for quite some time. The first mission to be flown in the Prometheus project was the Jupiter Icy Moons Orbiter, or JIMO. The goal was to explore the three icy moons within the context of the Jupiter system. It was a very ambitious project. Well, earlier this year JIMO was cancelled. But it looks as though this coming year there will be approval for a geophysical orbiter for Europa. The initial steps for getting that spacecraft underway are being considered now. Europa is a very high priority for exploration, and in recognition of that priority, this mission is likely to happen.
Why are we so interested in Europa? When we talk about astrobiology, we consider the three ingredients for life: water, the right chemistry, and energy. Their presence doesn’t mean that the magic spark of life ever happened, but those are the things that we think are required for life. And so, as I outlined, all three of Jupiter’s icy moons are potential targets. But Europa is the highest priority, because it seems to have the maximum internal energy.
So, of course, first we would like to know: Is there an ocean, yes or no?
Then, what’s the three-dimensional configuration of the icy crust? We know that organisms can live in fractures and cracks in Arctic ice. Such cracks are likely to be present on Europa, too, and could be niches that are of high interest for astrobiology.
Then we want to map the organic and inorganic surface compositions. We see in the data that exist today that the surface is heterogeneous. It’s not just pure ice on the surface. There are some areas that seem to be richer in non-ice components than other places. We want to map that material.
We also want to map interesting surface features and identify the places that are most important for future exploration, including landers.
Then we want to understand Europa in the context of the Jupiter environment. For example, how does the radiation environment imposed by Jupiter affect surface chemistry on Europa?
Ultimately, we want to get down on the surface, because there are a number of things that we can do only from the surface. We have a great wealth of data from the Galileo mission, and hope to have even more from the potential Europa mission, but it’s remote-sensing data. Next, we want to get a lander onto the surface that could make some critical ground-truth measurements, to place the remote-sensing data into context. And so within the scientific community, we feel that the next mission to Europa and the Jupiter system ought to have a landed package of some kind. But whether this will actually happen or not, stay tuned!
Original Source: NASA Astrobiology